Abstract:
The Dead Sea is the lowest spot on Earth. It is a closed saline lake located in the middle of the Jordan Rift
Valley between Lake Tiberias and the Red Sea. Its major tributaries are the Jordan River itself and the Dead Sea side wadis. The Dead Sea has a unique ecosystem and its water has curative, industrial and recreationalsigni®cance. The level of the Dead Sea has been continuously falling since the early 1930s at an average rate of 0.7 m per year. The water level, as of February 1998, is about 410.9 m below mean sea level. In this paper, a water balance model is developed for the Dead Sea by considering different hydrological components of this water balance, including precipitation, runo€, evaporation and groundwater ¯ow. This model is calibrated based on historical levels of the Dead Sea. Di€erent scenarios are investigated, including the proposed Dead Sea±Red Sea Canal. This project is supposed to halt the shrinking of the Dead Sea and restore it to pre-1950 levels in the next century. Copyright # 2000 John Wiley & Sons, Ltd. KEY WORDS Dead Sea; Jordan River system; water balance; salinity of the Dead Sea; Dead Sea±Red Sea Canal; Dead Sea levels; water in the Middle East
INTRODUCTION
The Dead Sea (DS) is a closed lake located in the middle of the Jordan Rift Valley between Lake Tiberias
and the Red Sea (Figure 1). The DS and its rugged eastern and western escarpments are part of the great
tectonic rift that extends from the Middle East to Africa. Historically, the DS may be divided into two water bodies, the northern and southern basins. The two bodies are separated bya small peninsula called the Lisan. The northern basin is a deep wide lake, whereas the southern one is very shallow. In the late 1950s, the level of the DS was 395 m below sea level (b.m.s.l.) and its length was about 80 km, with an average width of 17 km. The total surface area of the DS was then about 1000 km2, of which the northern basin constituted about 757 km2 and the southern basin about 240 km2.The maximum depth of the water was about 400 m in the northern basin and about 10 m in the southern basin (Abed, 1985). Similarly, in 1982, the level of the DS was 400.5 m b.m.s.l. and its total surface area was about 800 km2, of which the northern basin constituted about 740 km2 and the southern basin constituted about 60 km2. This implies that the shrinkage in the total area of the DS is very pronounced in the southern basin and not so in the northern basin (Abed, 1985). According to the Arab Potash Company (APC), the water level of the DS is 410.9 m b.m.s.l. as of February 1998, with a surface area of 645 km2 (APC, 1998). The DS surface is the lowest point on Earth. It is by far the most salty sea on Earth. Its salinity is about 345 g/l which is about 10 times the salinity of oceans and 50% greater than the salinity of the Great Lake in
Utah (Abed, 1985). Water ¯ows from the northern tributary of the Jordan and the Yarmouk rivers as well as
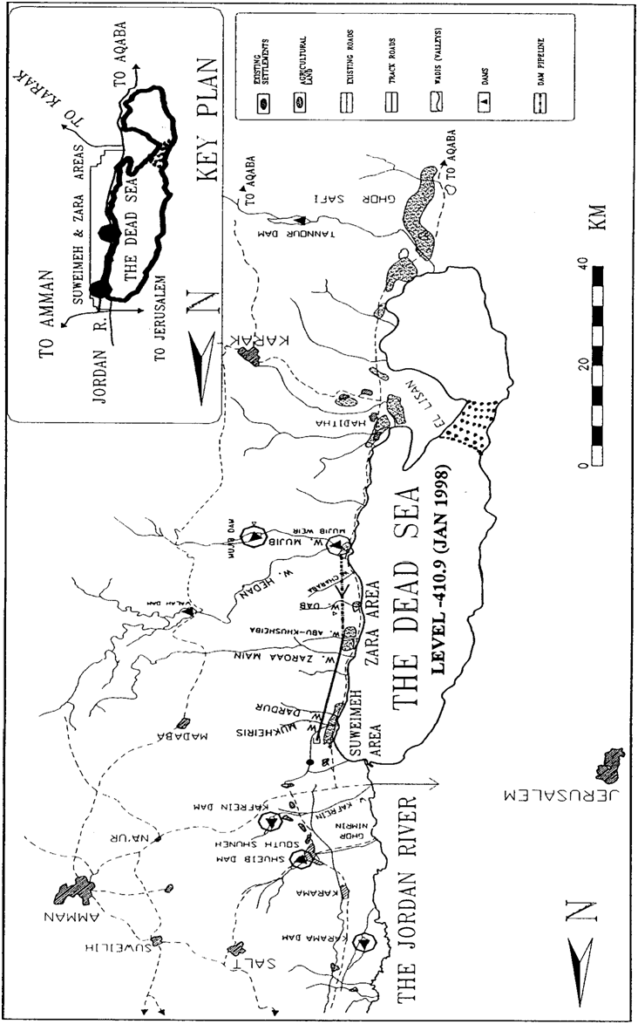
from side wadis on the east and west escarpments of the Jordan River and ®nally drains to the DS. The DS shores are shared by three communities, the Jordanians, the Israelis, and the Palestinians. Figure 1 shows a general location map of the DS and some of its side wadis and the lower Jordan River. The DS has much economical, historical curative and environmental signi®cance in the region. It also possesses a unique ecosystem and cultural heritage. Most of the available water resources that used to feed the DS are fully utilized by the riparian countries. Israel is transferring about 400 MCM (million cubic metres) a year through their national water carrier from the Jordan River basin to coastal areas on the Mediterranean Sea and the Naqav area in the south. Jordan is using about 40 MCM a year to supply Amman with drinking water from King Abdullah Canal originated mainly from the Yarmouk (WAJ, 1996). In addition, extensive local use of water for domestic and irrigation purposes has substantially decreased other water in¯ows to the DS. The historical in¯ow of the Jordan River at its entrance to the DS was about 1870 MCM in the late 1920s. This ¯ow dropped substantially to about 160 MCM per year in the early 1990s (Salameh, 1996). This drop can be attributed to natural decrease in ¯ood ¯ow as well as to excessive human activities upstream of the DS. Recent studies estimate that the average ¯ow in the Upper Jordan River system is about 1300 MCM peran num. About 47% is abstracted by Israel, 22% by Jordan, 16% by Syria and 2% by Lebanon. Only 13% of the mean annual ¯ow in the Jordan River System reaches the DS (JRV, 1996a). Most of this water is return ¯ow from the irrigation activities on the east and west sides of the Jordan River. The salinity of this ¯ow has substantially increased to reach about 15000 ppm. The subtropical climate of the DS area, as well as the industrial activities of the potash mining on the east and west coasts of the DS, make the evaporation rate about 1.5 m per year. It can be seen that the historical changes are substantial and this decline poses a serious risk to the DS ecosystem (EcoPeace, 1996).
WATER QUALITY OF THE DS
With the high temperature of the Dead Sea region, water continuously evaporates into the air, leaving a high salt concentration in the lake. This has produced one of the saltiest lakes in the world with an average salinity of 345 g/l which is about 10 times greater than that of the oceans. The water of the DS can be divided into two major layers, the surface top layer and the lower bottom layer. The top layer covers a thickness up to 100 m of the depth, and the bottom layer extends from the 100 m depth
to the DS bottom, which is at about 400 m. The properties of these two layers are di€erent in terms of
temperature, salinity and density. The salinity of the bottom layer is about 10% greater than the salinity of
the top layer. Similarly, the water density of the bottom layer is about 2±3% greater than the salinity of the top layer. Although the salinity of the DS water is high compared with other water bodies in the world, its mineral composition is also quite unique. As shown in Table I, it has a very high concentration of bromide, chloride, calcium, magnesium and sodium. The ratio of bromide to chloride concentration in DS water is about 1:35,
Table I. Chemical composition of the Dead Sea water (Abed, 1985)
Constituent | Average concentration (mg/l) |
Chloride | 212400 |
Bromide | 5120 |
Bicarbonate | 220 |
Sulfate | 470 |
Sodium | 39150 |
Potassium | 72б0 |
Calcium | 1б8б0 |
Magnesium | 50б50 |
comparedwiththeratioinoceanwaterof1:1000(Abed,1985).About11traceelementsarefoundinDS
water. These elements include lithium, manganese, strontium, cobalt, cadmium, lead, zinc, nickel, uranium
and iodine.The acidity of the DS water ranges from pH 6.1 to 6.7, with an average of 6.4, compared with ocean water, which has an average pH of 8.2. This means that the DS is more acidic than ocean water.
The location of the Dead Sea, its water salinity and mineral composition make ita natural source for
medical treatment sinceit contains a unique array of salts, enriched with magnesium, potassium,calcium
and bromine and relatively high concentrations of trace elements.Ithasahighspeci®cgravityofabout1.25
whichyieldsgreaterbuoyancy,makingiteasyto¯oatonitssurface(KlienandFlohn,1987).
FLOW IN THE JORDAN RIVER SYSTEM UPSTREAM OF THE DS
The water balance of the DS is dependent on the¯ows from the Jordan River system up stream of the DS
area,which are collected mainly by the lower part of the Jordan River, andthe¯owin the side wadis raining
directly totheDS(Figure1).Surface water¯owandabstractionfromeachsub-basinintheJordanRiver
the system has been evaluated and excess run €isrouted downstream through this system to estimate the net runo€ to the DS itself.
Two cases are considered in this analysis.The®rst is based on conditions before the Treaty of Peace
between Jordan and Israel water allocations(signedin1994),while the second case accounts for the treaty
allocations.Table II show as summaryof¯owsineachsub-basin with out the Treaty of Peace allocations,
whereas TableIII shows the same analysis with the Treaty of Peace allocations.The net estimated water¯ow
to the DS itself from the Jordan River system is found to be175MCM per year with out the Treaty of Peace
allocations and 60MCM per year with the Treaty of Peace allocations(JRV,1996a).This analysis shows the
impact of theTreaty of Peace allocations on the up stream into the DS.Thisin¯ow falls from13% of the
Table II. Annual water balance of the Jordan River system without Treaty of Peace allocations (JRV, 199бa)
Sub-basin (1) | Surface runoff and inflow (MCM) (2) | Total abstraction (MCM) (3) | Net flow balance (MCM) (4) |
Upper Jordan (North of Lake Tiberias) | б50 | 170 | 480 |
Lake Tiberias | 490 | 410 | 80 |
The Jordan Below Tiberias | 80 | 50 | 30 |
Yarmouk River | 475 | 350 | 125 |
Side wadis of the Jordan | 180 | 1б0 | 20 |
Lower Jordan | 175 | − | 175 |
Total | 1315 | 1150 | 175 |
Table III. Annual water balance of the Jordan River system with the Treaty of Peace allocations (JRV, 199бa)
Sub-basin (1) | Surface runoff and inflow (MCM) (2) | Total abstraction (MCM) (3) | Net flow balance (MCM) (4) |
Upper Jordan (North of Lake Tiberias) | б50 | 170 | 480 |
Lake Tiberias | 5б0 | 490 | 70 |
The Jordan Below Tiberias | 70 | 50 | 20 |
Yarmouk River | 405 | 375 | 30 |
Side wadis of the Jordan | 180 | 1б0 | 20 |
Lower Jordan | 70 | 10 | б0 |
Total | 1315 | 1255 | б0 |
total flow in the Jordan River system without the Treaty of Peace allocations to about 5% with those
allocations.
THE WATER BALANCE MODEL OF THE DS
A water balance model is developed for the DS that uses time-series analysis for in¯ows, out¯ows and
evaporation to simulate the conditions of the DS over time. This model was calibrated using historical
in flows and surface water levels of the DS. The DS annual water balance analysis incorporates the following components of in flows and outflows.
1. Surface in¯ows from the Jordan River System, average values are shown in Tables II and III;
2. Surface in¯ows from eastern Wadis that ¯ow directly to the DS. Eastern wadis include Hisban, Mujib,
Wala, Karak, Hassa, Zarqa Ma’in and Zara springs. The average annual ¯ow is about 34 MCM in Hassa, 18 MCMin Karak, 83 MCM in Mujib and Wala and 23 MCM in Zarqa Ma’in (Salameh, 1996).
3. Surface in¯ows from western wadis that ¯ow directly to the DS. Western wadis include Tekoa, Himan,
Arugot, Admon andRahaf. No published records of ¯ow in these wadis are available but the total ¯ow from
the western wadis is estimated to range from 20 to 120 MCM (Anati and Shasha, 1989; Klien, 1990).
4. Rainfall on the DS itself. The average annual rainfall over the DS is about 100 mm. The volume of this rainfall is estimated based on the surface area of the DS which is a function of its water level.
5. Evaporation from the DS surface itself. Many studies have addressed DS evaporation, which is a function of its salinity (e.g. Abed, 1985; Stanhill, 1994; Cohen and Stanhill, 1996). Stanhill (1994) developed
an algorithm to estimate the DS evaporation using the standard energy (heat) balance approach for a unit
area of the DS. His approach lists all the sources and sinks of thermal energy and leaves the net heat available for evaporation as the only unknown. His algorithm evaluates the evaporation rate as a function of the DS surface density. He suggested that, during the past 40 years, there has been a relative decrease in the DS evaporation rate due to the increase in its salinity. He proposed a linear model that correlates the annual evaporation rate and the DS surface density. This relationship is given by (Stanhill, 1994)
E 4701 2926rs
where E is the annual evaporation rate (mm/yr) and rs is the surface density of the DS water in g/cm3. This relationship accounts for 98% of the variation between annual evaporation from salt-free water and from the salt-saturated water of the DS after 1981 (Stanhill, 1994). The measured data used to calibrate Stanhill’s work were for the period 1983±1987. When comparing Stanhill’s results with other available evaporation studies that used the same approach (e.g. Abed, 1985), it was noted that the Stanhill model considerably underestimated the evaporation from the DS. In fact, Stanhill himself noted that his model underestimated evaporation considerably for the periods 1942±1946 and 1979±1981 (Stanhill, 1994). Stanhill’s results were checked against in¯ows and surface density measurements during the 1930s (which were not covered by Stanhill data), and it was observed that his model produced a negative water balance during this period (JRV, 1996a). Although this negative water balance may be due to other factors in the water balance, the effects of these factors were checked and eliminated. Thus, according to JRV studies, the most reasonable explanation is the underestimation of the evaporation rates in the Stanhill approach. In addition, Stanhill estimated an annual evaporation rate of 1.05 m, contrary to other studies which indicate an annual evaporation rate ranging from 1.30 to 1.54 m (Abed, 1985). These points suggested that the Stanhill algorithm underestimated evaporation by about 35% (JRV, 1996a). This result explains the negative water balance according to the Stanhill algorithm during the 1930s. Therefore, a modi®cation factor of 1.35 was used by the JRV study to account for this underestimation in the
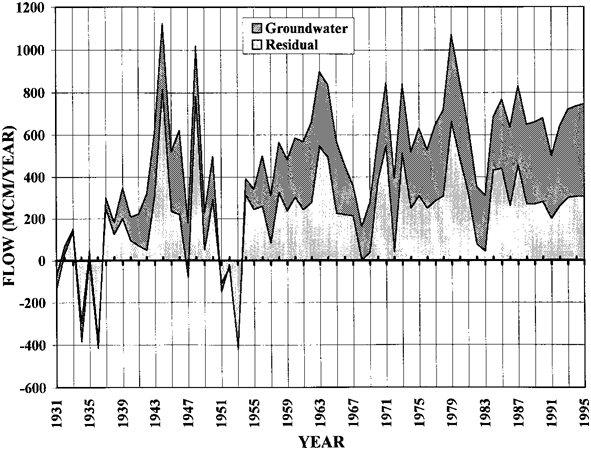
Figure 2. Residual flow time series and groundwater inflow time series for the DS
DS evaporation by the Stanhill algorithm (JRV, 1996a). The JRV modi®ed relationship between the surface
density and evaporation rate analogous to Stanhill may be expressed as
E= 6346-3950rs
where E is the annual evaporation rate (mm/yr) and rs is the surface density of the DS water in g/cm3.
6. Abstractions from potash mining and works on the east and west sides of the DS. Production ®gures
from the Arab Potash Company (APC) on the east side of the DS are currently 1.8 million tons and are projected to increase to 2.2 million tons by 2001. Similarly, the Israeli Potash works production is about
2.3 million tons per year. Average brine abstraction rates from the DS are estimated to be 118 cubic meters of brine for a ton of production. Thus, the total future abstraction rate is about 531 MCM/year according to plans. About 40% of the abstraction returns to the DS as brine return ¯ows (about 213 MCM/year).
7. Estimated groundwater in¯ows. This component is the most difficult to estimate explicitly. In this
model, it is estimated based on the residual in¯ow needed to balance the water budget of the DS. The residual in flow is de®ned as the sum of all out¯ows (including evaporation) minus the sum of surface inflows and direct rainfall of the DS. It can be seen that the residual in¯ows have increased over time as the level of the DS has fallen. This indicates that the residual in¯ow is a function of the groundwater in¯ow to the DS. The residual in¯ows’ time series was highly correlated to the groundwater water in¯ow time-series, as shown in Figure 2. This analysis agrees with other independent observations in Jordan that groundwater in¯ows to the DS have increased over time as the DS levels have fallen (JVA, 1996). However, no detailed aquifer studies are available to quantify the groundwater in¯ows in the DS region. Thus, the residual approach is the best available one which can be used to quantify the groundwater in¯ows. It must be stressed here that this estimation of groundwater in¯ows may be influenced by the evaporation rates adopted in our analysis. The groundwater component analysis cannot be calibrated; however, the residual approach presented in Figure 3 does provide a reasonable indicator of the order of magnitude of the groundwater in¯ows (JRV, 1996a).
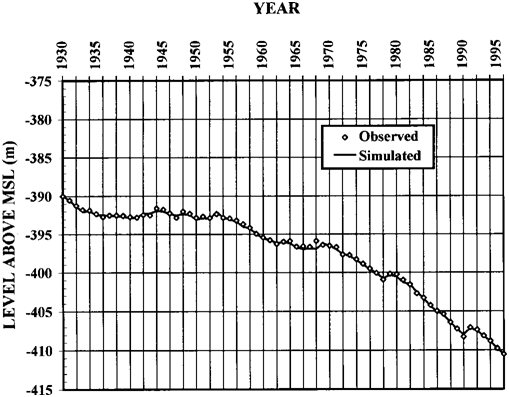
MODEL CALIBRATION BASED ON DS LEVELS
The water balance model developed for the DS was calibrated based on historical water level records since the early 1930s and refers to the same time of the water year. The level of the Dead Sea was reported to be around 398 m b.m.s.l. in 1810 AD. This level ¯uctuates considerably as reported as 388.8 m b.m.s.l. in 1895 and 390 m b.m.s.l. in 1930. Beyond 1955 a continuous decrease in the level of the DS, except for a very few wet years, has occurred. The level reached about 410.9 m b.m.s.l. by February of 1998, where the surface area of the DS at this level is about 645 km2. However, the design level considered for most projects on the DS is 395 m b.m.s.l. which corresponds to an area of 738 km2. This level was taken to accommodate any future development schemes in the Dead Sea area like the Red Sea±Dead Sea Canal. Figure 3 shows the historical data of water levels of the DS compared with simulated levels using the water balance model and referring to the same time of the water year. The di€erences are quite small and thus the water balance model does give a relatively good ®t to historical data. Therefore, such a model is useful and adequate for planning of any water project related to the DS.
WATER BALANCE OF THE DS UNDER DIFFERENT SCENARIOS
The Treaty of Peace signed in 1994 between Jordan and Israel a€ected the water use and allocations for
di€erent entities in the Jordan River system. As shown in Table II, the net in¯ow to the DS from the Jordan
The river system falls from 175 MCM per annum (about 13% of the total ¯ow in the Jordan River system) without the treaty to 60 MCM(about 5%) after the treat. This decrease is due to the fact that Jordan is entitled to use 20 MCM of the ¯oodwater from the Jordan River and to store another 20 MCM of excess ¯oodwater in Lake Tiberias during winter for its use in the summer. The abstraction by Jordan from the Yarmouk under the treaty is increased by 25 MCM. Another 50 MCM of additional sources that Israel agrees to provide to Jordan may also come from the Jordan River system upstream of the DS.
The RedSea±DeadSeaCanal(RSDSC)is amajor project in the JRV which is made possible by the peace
treaty between Jordan and Israel. This project will connect the Red Sea near Aqaba to the southern end of the Dead Sea by a conveyance system located in the Wadi Araba between Israel and Jordan. A schematic diagram showing the settings of the RSDSD is shown in Figure 4.
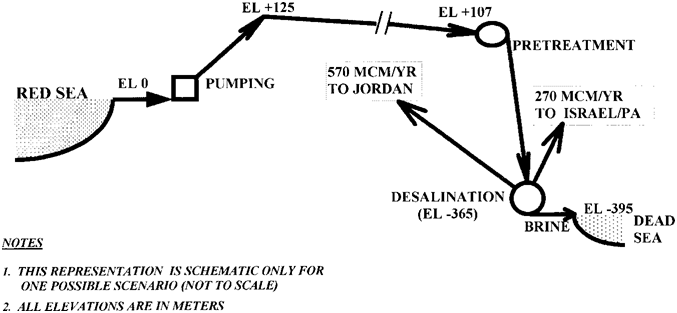
The main objectives of this project are to provide a sustainable source of fresh water and to halt the decline in the DS level. Various alignments are proposed and evaluated at this stage taking into account topographical, geographical, environmental and operational aspects. The total length of the conveyance system ranges from 196 to 229 km depending on the alignment. Major components of this conveyance system include a rising main, tunnel, canal, penstock, siphon and other control and regulating structures. A pumping lift station is needed near the Red Sea end to provide a head of 200 m of water to overcome topography in the ®rst few kilometers of the system for some proposed alignments. Thus, a total available hydrostatic head near the Dead Sea end ranges from 331 to 545 m of water, depending on the alignment and the pumping lift provided at the Red Sea end. The capacity of this conveyance system was studied under di€erent diversion flow rates ranging from 40 to 70 m3/s (JRV, 1996b). A desalination plant is proposed to produce fresh water using the reverse osmosis method. The estimated annual fresh water production of this plant is a function of the conveyance system capacity and it can reach 850 MCM, depending on the diversion capacity. It is expected that two-thirds of this production will go to Jordan and the rest will go to both Israel and the Palestinian side (JRV, 1996b). The e€ects of four scenarios, depending on the capacity of the RSDSC, are evaluated in the DS water balance model for the next 50 years. The ®rst scenario assumes a diversion capacity of 70 m3/s. It shows that the DS will restore its design level of 395 m in about 40 years. The second scenario assumes a diversion capacity of 60 m3/s and shows a level of 400.5 m in about 40 years. The third scenario assumes a diversion capacity of 50 m3/s and shows a level of 406.6 m in about 40 years. The fourth scenario assumes a diversion capacity of 40 m3/s and shows that the DS will reach a level of 413.3 m in about 40 years. A ®fth scenario assumes no project (no RSDSC) and the model shows that the DS level will fall to 444.4min about 40 years. All these scenarios are based on a DS level of 408.5 m based on 1995 observations. Table IV compares the predicted levels of the DS under di€erent scenarios in about 40 years, starting from a level of 408.5, as of 1995.
Year | 1995 | 2005 | 2015 | 2025 | 2035 |
Scenario 1 Scenario 2 | —408.5 | —41б.8 | —414.9 | —403.5 | —395 |
Cost of the RSDSC desalinated water
The cost of the RSDSC production of fresh water was analyzed assuming a conveyance diversion capacity
of 60 m3/s. The estimated total project cost is about US$ 5 billion at 1996 prices (JRV, 1996b). The estimated cost of fresh water production ranges from US$1.3to1.5 per cubic metre. This cost is still relatively high and cannot be a€orded by most users in the region considering their economic conditions.
DISCUSSION AND CONCLUSIONS
The surface water level of the DS has been falling continuously at a rate of 0.7±0.8 m per year in recent years. This decrease in its water level is due to excessive water development and abstraction from the main tributaries of the DS. The potash mining activities on both sides of the DS have increased the rate of water loss from the DS. This reduction adversely a€ects the unique environmental setting in the DS area
(EcoPeace, 1996). The in¯ow from the Jordan River to the DS was about 175 MCM, this number fell to 60 MCM after the Treaty of Peace water allocations between Jordan and Israel. A water balance model using time-series analysis was developed for the DS considering all in¯ows and out flows from the system. This model was calibrated using historical-level measurements for the DS. The RSDSC is a major project that will a€ect the DS water balance when implemented. The main objectives of this canal project are to provide a sustainable source of fresh water to the region and to halt the decline in the DS levels.
Based on the above analysis the following conclusions can be drawn.
1. The continuous decline in the DS water levels is an environmental disaster which adversely a€ects
the unique ecosystem of the DS and its surroundings.
2. Human activities and developments in the Jordan Valley upstream of the DS accelerate the water level
decline in the DS. The Treaty of Peace water allocations between Jordan and Israel have decreased the in flow to the DS from the Jordan River from 175 to 60 MCM per year.
3. Human activities and developments in the Jordan Valley upstream of the DS accelerate the water level
decline in the DS. The Treaty of Peace water allocations between Jordan and Israel have decreased the in flow to the DS from the Jordan River from 175 to 60 MCM per year.
4. The RSDSC will eventually be a beneficial project to the DS. It will halt the decline in the water levels
of the DS and will restore the historical water levels of the DS (prior to 1930s) in about 40±70 years from the commissioning date, depending on the canal capacity.
5. The waters of the DS are unique in their properties. They can be utilized as a major attraction in the
region for industrial, tourism and curative activities. Both the east and west coasts of the DS are undergoing major tourism developments.