Omar Al Hattamleh1 & Hussein Aldeeky1 & Samar Rabab’ah2 & Madhar Taamneh3
Abstract
Dead Sea salt, a benign chemical material vastly available in Jordan, could be used to improve the characteristics of expansive subgrade soil and to reduce carbon dioxide emissions resulting from traditional stabilizers such as cement. To achieve this purpose, expansive clayey soil obtained from the Al-Azraq Basin in the eastern part of Jordan was treated by mixing it with a Dead Sea salt solution. Results showed that using Dead Sea salt as a solution additive, the plasticity index (PI), free swell (FS), and optimum moisture content (OMC) decreased. On the other hand, the maximum dry density (MDD) and the unconfined compressive strength (UCS) noticeably increased, continuing to strengthen with increased curing time. Additionally, with wetting-drying cycles to OMC, FS was reduced, reaching equilibrium value with fewer cycles. However, with the wetting drying cycles to shrinkage limit, FS slightly increased. In conclusion, using Dead Sea salt as a stabilization additive markedly enhanced the engineering properties of the expansive clay used as subgrade soil.
Keywords DeadSeasalt(DSS)solution.Atterberglimits.Freeswell.Unconfinedcompressivestrength.Maximumdrydensity .Wetting-drying cycles
Introduction
Expansive soil is characterized by its susceptibility to volumetric changes when free water is available, increasing in volume upon wetting and shrinking if it dries out. The
OmarAlHattamleh Hattam@hu.edu.jo
Hussein Aldeeky aldeeky@hu.edu.jo
Samar Rabab’ah srrababah@just.edu.jo; srababah@hu.edu.jo
Madhar Taamneh mtaamneh@yu.edu.jo
1. Civil Engineering Department, College of Engineering, Hashemite University, P.O. Box 150459, Zarqa 13115, Jordan
2. Civil Engineering Department, College of Engineering, Jordan University of Science & Technology, P.O. Box 3030, Irbid 2210,Jordan
3. Civil Engineering Department, Hijjawi Faculty for Engineering Technology, Yarmouk University, Irbid, Jordan
swelling and shrinkage of expansive soil depend on the type and the amount of clay minerals, dry density, moisture content, loading condition, and the total cations (Al-Shameri et al. 2013; Salima and Abdelhak 2013; Yilmaz and Marschalko 2014; Jayasree et al. 2015). This type of soil is found worldwide, especially in arid and semi-arid areas. In wet seasons, this soil will heave, causing the lifting of lightweight structures, while in dry seasons, it will shrink, causing the collapse of the structures (Al-Rawas et al. 2005; Turkozetal.2014; Mahamedi and Khemissa 2015). This phenomenon causes damage to lightweight structures, roads, and drinking water networks (Al-Rawas et al. 2002; Shi et al. 2002; Fredlund 2006; Parfitt et al. 2011; Ozer et al. 2012; Karunarathne et al. 2012; Fouzan and Dafalla 2013; Salahudeen et al.
2014). For this reason, extensive studies have been performed to improve expansive soil properties in terms of reducing swell and increasing strength (Murty and Praveen 2008; Fouzan and Dafalla 2013; Salahudeen et al. 2014). Marginal or so-called soft rock such as carbonate rocks and marlstones
are problematic in their behavioral nature. Though they are strong enough when dry, they are weak when wet. Shahri and his research team extensively evaluated the UCS of such materials, developing a model to predict their mechanical strength as well as predict clay sensitivity in a landslide-prone area (Shahri et al.2016; Shahri2016; Asheghi et al.2019). Toimproveexpansivesoils, three kinds of improvement methods have been adopted,namely mechanical, physical, and chemical (M.Al-Akhrasetal.2008;Hasanetal.2015;
Rahmatetal.2015). Mechanical approaches using compaction, preloading,soil replacement,and soils enforcement have been investigated by many researchers (Yilmaz2006;
Viswanadhamet al.2009;ChegenizadehandNikraz2011; Shillitoet al. 2014;Chuet al. 2014;SchanzandElsawy
2015).Chemicalmethodsusingmaterialssuchas fly ash, cement, lime,or gypsum,and recycled materials such as slag, scrap tires, glass, bitumen,and salt have been investigated (Çokça2001; Petersen et al. 2003; Al-Rawaset al. 2005; Sezer et al. 2006; Kumar et al. 2007; Yilmaz and Civelekoglu2009; Solanki andZaman2010; Al-Mukhtar etal.2010; Kolayetal.2011; Ouetal.2011; Sarkar et al. 2012;Mansoetal.2013;Sarideetal.2013;PalandGhosh
2014;Zhaoet al. 2014;KhemissaandMahamedi 2014; Sivapullaiahand Jha2014; Schanz andElsawy2015;
AldeekyandOmarAlHattamleh2017).Soil stabilization using physical methods such as wet-dry cycles and thermal treatment also have been investigated(Chen1988;Abu Zreigetal.2001).Turkozetal. (2014) usedMgCl2 to study its effect on expansive oil properties; the results revealed an improvement in the engineering properties after using the MgCl2 solution. This result agreed with prior research (Goodrichetal.2009; Jianlietal.2009).Aftera7-day curing period, thestrengthofthepeattreatedwith6%MgCl2was6 timesthatoftheuntreatedpeat(Latifietal.2016).
Lajurkaretal. (2016)useda1.5and2%concentrationof CaCl2toimproveblackcottonsoil; theresultshowedapos
active effect by improving the strength and reducing the swelling behavior.Theimprovementofexpansivesoilwitha5% concentration of CaCl2 resulted in decrease in the PI,OMC, and FS, and a boost to the MDD and the UCS(Zumrawiand Eltayeb2016).DubeyandJain(2015) found that the addition
of an 8% concentration of NaCl to black cotton oil decreased the California Bearing Ratio (CBR) values and shear strength parameters, also decreasing the free swell. Based on Shuklaet al. (2014), the addition of 17mg/L concentration of sea salt to black cotton oil resulted in a drop in the plasticity and free swell indices,and an increase in the dry density.Ivasuc(2012) evaluated the influence of seawater on expansive clay, showing that the addition of sea water reduced the PI, and this reduction appeared clearly in bentonite soil.Yukselen-Aksoyetal. (2008) showed that the soil on sistency and compressibility were affected by the addition of the Aegean sea water when liquid limits exceed110%except for Nabentoniteclayey soil. Chittaranjanetal. (2011)showed that using seawater result dinar eductionof10.9%
in PI for clayey soil,while OMC,MDD, UCS, and thefts index increased by 30%,7.6%,20%, and 6.7%,respectively.
Materials and method
Soil
In this research,expansivesoilwasobtainedfromAl-Azraq Basin.Al AzraqBasin(110kmtothenortheastofAmman, Jordan)ispartoftheeasterndesertinJordan,whichisclassi
fiedasanaridregionwitharound50-mmannualrainfall.The
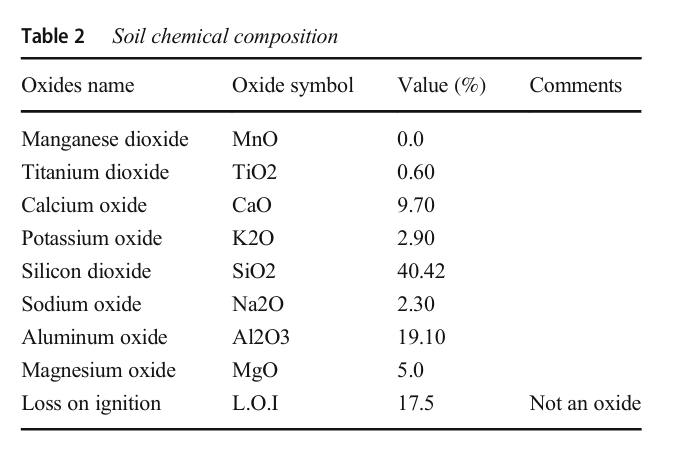
sample was collected by manual excavation in disturbed conditions about 1m from the ground surface. Al-Azraqclaysoilis composed of mixed layers of illite/smectite, Kaolinite, palygorskite, and discrete illite(Nawasreh2001; Hawari and BanyYaseen2012).To classify the soil sample, grain size is tribution, LL,PL, specific gravity, standard compaction,and pH tests were performed.The American Society for Testing and Materials standards(ASTM)were used to conduct all the experimental tests.Table 1 shows the physical properties of the soil studied.BasedontheUnifiedSoilClassificationSystem(USCS) (ASTMD2487-172017),the soil was classified as fat clay (CH) (ASTMD2488-172017).Soil chemical composition,as obtain ed using standard X-ray fluorescence spectroscopy(ASTM
E1621-132013), is provided in Table 2.
Dead Sea salts
Fifty kilometers long and 15km wide, the Dead Sea lies in the Jordan Rift Valley about 430.5mbelowthe sea level. The Dead Sea is a Salt Lake with 35% salinity, which is 9.6 times the salinity of oceans.Bulk samples of DSS were crushed and dissolved in distilled water to obtain a solution with 350 g/1 kg salinity. The DSS solution properties obtained using standard X-ray fluorescence spectroscopy are shown in Table 3. Sodium chloride (NaCl) in the DSS solution is about 30%, while NaCl in the salt of most oceans and seas is about 85%. Based on the abovementioned constituents of DeadSea salt, the richness of divalent cations such as Mg2+ andCa2+, and their high concentrations,DSS can be used as a green chemical treatment supplement instead of lime and/or cement, which contributes to the increase of global warming.
Sample preparation
The soil sample was dried out at 105 °Cfor 24hand then sieved with an o. 4sieve.Variable amounts of the
DSSsolutionwith35%salinity(0, 3, 6, 9, and12%by dry soil mass) were mixed manually with the soil to
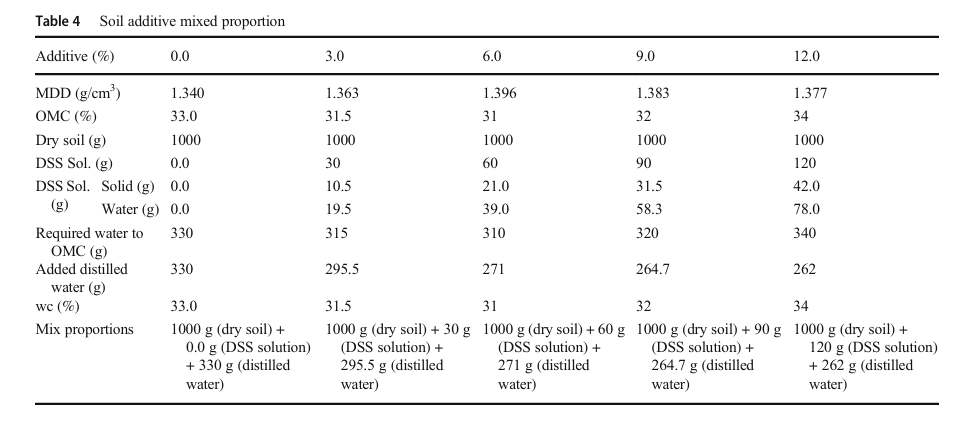
obtain a homogenous mixture. Soils with additives were kept in closed plastic bags for 24 h. The standard com paction test (ASTM D698) was performed at each additive percentage. FS and UCS samples were prepared at the OMC and MDD at every additive percentage. The mixes’ proportions utilized in the current study are shown in Table 4.
Results and discussion
Liquid and plastic limits
LL and PL tests were conducted on soil samples with/ without additives. LL, PL, and PI results are presented in Fig. 1. Both LLD and PL decreased as the additive contents increased; consequently, a reduction in PI occurred. As can be seen in Fig. 1, beyond 9% of the additive, insignificant changes were observed for both LL and PL. These reductions in LL and PL are attributed to the decrease in diffuse double-layer thickness. Furthermore, increasing salt concentration and cations valence decreases the interparticle repulsion, and as a result, the particles move freely in lower water content.
Dry density-water content relationship
The standard compaction test (ASTM D698) was conducted to determine the relationship between the MDD and OMC on soil samples with/without additives, as shown in Fig. 2. The results show that the addition of a DSS solution (up to 6%) decreased the OMC and increased MDD. An explanation for these results is that there is a lessening in the diffuse double layer thickness and higher cations valance as the salt content increases, in addition to the soil particle flocculation. The 6% DSS solution gave the highest MDD and the lowest OMC. However, later, an increase in OMC and a decrease in MDD were observed
Free swell percentage
Soil with/without additives was compacted to the MDD and OMC in a mold with a diameter of 70 mm and a height of 20 mm to study the effect of the DSS solution on the volumetric changes of expansive clay. The soil specimens were placed in the odometer cell with double drainage; after adding distilled water, the soil specimen was allowed to freely swell under a surcharge pressure of 7.0 kPa. This procedure was adopted according to ASTM D4546-14 (2014). Figure 3 shows varies FS percentage with time for the treated and untreated soil samples. A decrease in the FS percentage with the increase in the additive content was observed; the maximum FS percentage decreased from 10.4% at 0%additive to 3.35% at 12% additive
Unconfined compressive strength
Each UCStest sample was remolded to the MDD and the OMC. The prepared soil samples were wrapped and sealed in plastic bags and placed in a sealed container at room temperature for 1 day, 7 days, and 28 days to allow for the DSS additive and the curing time on specimens’ strength to be assessed. A similar
procedure was used by Turkoz et al. (2014) in evaluating the effect of magnesium chloride solution on the engineering properties of expansive soil. The reported UCS results were tested according to ASTM D2166 / D2166M-16 (2016). UCS values after a 1-day curing time are displayed in Fig. 4.The results show
that when the DSS content was increased to 6%, the UCS strength values increased, and then slightly decreased after that. UCS values increased from 133.6 kPa at 0 % additive to 178.4 kPa at 6% additive, while the strain at failure decreased from 4.34 to 3.95%, respectively. After 6% of the DSS additive, the values of UCS decreased as a result of the increase in the positive charges in the clayey particle surfaces and as a consequence, the repulsion between soil particles increased (Latifiet al. 2014; Turkozetal.2014). Figure 5 shows the effect of DSS content on the UCS at different curing times. From Fig. 5, the increase in the curing time resulted in an increase in the UCS values. At 6% additive content, the UCS increased from 178.4 kPa after a 1-day curing time to 194.4 kPa and 234.6 kPa for 7- and 28-day curing times,
respectively. Similar observations of the gaining of strength with time (thixotropy) for treated active clayey soils were previously reported by other researchers (Latifi et al. 2014; Turkoz et al. 2014). The increase in the UCS for treated expansive soil with the addition of salt is due to the recrystallization of the DSS in the pore spaces, which in turn increases the MDD (Turkoz et al.2014). Moreover, Tingle et al. (2007) observed that the addition of salt to soil tends to increase the pore water tension in the soil matrix, which in turn improves the shear strength of the treated soil according to the effective stress principle.
Effect of wetting-drying cycles on the free swell of 6.0% DSS-treated soil Drying to optimum water content (partial shrinkage)
To study the consequence of WD to the OMC (partial shrink age) on free swell (FS), two soil specimens with/without a 6% DSS additive were compacted to the MDD and the OMC. Following the procedure set by Li et al. (2015) to ensure that the specimens attained water content equilibrium, the specimens were sealed in plastic bags for 24 After that, the FS test was performed under 7 kPa of surcharge pressure. After the FS reached the maximum value, the water around the sample was drained using the drained bath provided in the consolidation cell. The mold was dismantled, but the specimen kept in the brass cell ring. Then, it was allowed to dry and shrink at a room temperature of 30 °C; the weight of the
sample was measured every 15 min until it reached the OMC. This was considered as 1 cycle. After reaching the OMC, the samples were allowed to swell by adding tilled water. This procedure was repeated for 6 cycles. Figure 6 shows the variation of water content versus elapsed time for soil samples with/without additives. The results show a decrease in water content with wetting
drying cycles in both samples with and without additives. The variations of swell and shrinkage versus elapsed time are shown in Fig. 7 The FS for soil without an additive decreased with WD cycles, with the maximum decrease occurring in the first cycle, and then the FS gradually decreasing until it reached equilibrium after four cycles. The trend of the reduction of FS with the cyclic application of wetting-drying reported here mimics the results previously reported by many other investigators (Basma et al. 1996; Abdullah et al. 1999; Guney et al. 2007; Aldaood et al. 2014; Estabragh et al. 2015). The WD cycles caused the fine clay particles to flocculate. The size of fine clay particles increased, and the specific surface area decreased due to large particle sizes, causing a reduction in the FS. In addition, the apparent voids were reduced during the WD cycles, which in turn reduced the ability to absorb more water during rewetting.
Soil with a 6% DSS additive showed the same trend in FS reduction with WD cycles, reaching the equilibrium after the third cycle. This reduction was modest when compared to soil specimen without an
additive, as shown in Fig. 7. A 6.0% DSS solution acted as a salinity agent flocculating the soil particles
and causing a reduction in the FS often within WD cycles; a similar result was obtained when using a salt
solution as pore water as reported by Abdullah et al. 1999; Lin and Benson 2000; Karimpour-Fard et al.
2011; Soltani and Raeesi Estabragh 2015.
Drying below shrinkage limit (full shrinkage)
The shrinkage limit (SL) of the soil without/with 6% DSS was measured according to ASTM D427-2004. The SL was 22% and 20% for 0% DSS and 6% DSS, respectively.Soil samples with a 6%DSS additive and without additives were prepared as previously outlined and permitted to dry below the shrinkage limit (ASTM D427). To ensure that the samples with a 6% DSS/without additives dried out below the
shrinkage limit, the water content was measured in both wet and dry states at each period as the aforementioned procedure in the previous section. The results in Fig. 8 show that the
water content during wetting cycles increased up to the fifth cycle in the soil sample without additives, while in the case of the soil with the 6% DSS additive, the water content increased up to the third cycle then started to decrease after that. During the wetting of the dried expansive soil, the pores filled with
water were subjected to two types of deformation, the elastic one is reversible upon drying while the other is inelastic(permanent) due to the loss of interparticle forces arising from meniscus water (Estabragh et al. 2017). The most inelastic pores increased in the first cycle; thus, when the pore fluid was distilled water (Fig. 8a), the water content increased until it reached the equilibrium water content, as pointed out by Hanafy (1991). On the other hand, when the DSS was used as pore fluid, some of the salts accumulated in these pores, leaving less volume for water to occupy. Below the shrinkage limit, the increase in the number of cycles increased the water content in the sample without the additive, but it decreased the
water content in the sample with the 6% DSS additive. The variation of FS with elapsed time is shown in Fig. 9. With the increase in the number of WD cycles in the soil sample without additives, FS gradually increased up to the fifth cycle. The increase in the number of cycles resulted in a decrease in shrinkage degree, with the largest decrease observed after the first cycle. Other researchers (Basma et al. 1996;
Mehri et al. 2017) observed a similar trend. An explanation for this trend could be that the free water evaporation during shrinkage increases the apparent cracks that are subsequently
filled during rewetting, resulting in an increase in the water content. For the soil sample with a 6% DSS additive, the FS slightly increased with WD cycles up to the third cycle, then slightly decreased, reaching equilibrium after the fourth cycle. Shrinkage cracks and a 15% reduction in sample diameter
were observed in the sample without a 6% DSS additive. Furthermore, the presence of high concentrations of double valence cations such as Ca2+ and Mg2+ (see Table 3) and the strong monovalence K1+ leads to the aggregation of soil particles (Sridharan and Allam 1982), decreasing the number of available pores filled with water, thereby decreasing the free
swell percentage (Fig. 9b).
Scanning electron microscopy analysis
Scanning electron microscopy (SEM) was conducted on soil samples with/without a 6% DSS additive after the sixth WD cycle to investigate the change in soil microstructure. As can be seen in Fig. 10, the soil sample without the additive shows fewer amounts of internal voids and a higher-density structure,
while the soil sample with a 6% DSS additive contains more internal voids and an aggregated structure because the 6% DSS additive flocculated and increased the soil particle size.
Conclusion
The physical and mechanical properties of subgrade soil stabilized with a DSS additive were studied. Expansive clay was treated with different amounts of a DSS solution. The DSS additive enhanced the most desirable properties of expansive clay evaluated in this study, specifically the tendency of volume changes upon wetting and drying. Based on the test results, the MDD increased and the OMC decreased with up to 6% of the DSS additive. However, increasing the additive content to 9 or12%slightlydecreased the MDD and increased the OMC. Moreover, UCSincreased asthe DSS additive content increased up to 6%, and then the UCS decreased with further increases in the additive content. Furthermore, the FS
percentage decreased from 10.4% at 0% additive to 4.45% at 6%additive content. With wetting-drying to OMC cycles, the FS was reduced in both samples with/without a 6% DSS additive but reached equilibrium with fewer cycles with a 6% additive. For wetting-drying below shrinkage limit cycles, the FS slightly increased in both soil samples with a 6% DSS additive and without additives. Lastly, the SEM image
showed that adding a 6% DSS additive increased the voids inside the soil structure as a result of flocculation and the increase in the size of the particles.