ZeevMa’or1,2,YigalHenis3,4,YaacovAlon4, Elina Orlov4, Ketil B. Sørensen5, and Aharon Oren6
From the 1Dead Sea Research Center, 86910 Dead Sea, Israel; 2Myers’ Laboratory for Skin Biochemistry and Biology, The Hebrew University of Jerusalem, 91904 Jerusalem, Israel; 3Faculty of Agricultural, Food and Environmental Quality Sciences, The Hebrew University of Jerusalem, Israel; 4Bactochem Laboratories, Ness-Zyona 74031, Israel; 5Marine Sciences Department, University of North Carolina at Chapel Hill, Chapel Hill, NC 27599, USA; and 6The Institute of Life Sciences, and The Moshe Shilo Minerva Center for Marine Biogeochemistry,
The Hebrew University of Jerusalem, 91904 Jerusalem, Israel
Our work was supported financially by a grant from the “Dead Sea Research Center”, a nonprofit organization recognized by the Israel Ministry of Science, which promotes scientific exploration of the Dead Sea area under the auspices of the Ben Gurion University of the Negev Report.
Correspondence
Zeev Ma’or
Dead Sea Research Center 86910 Dead Sea
Israel
E-mail: zeevi_m@ahava.co.il
Background The unique, black, hypersaline mud mined from the Dead Sea shores is extensively used in mud packs, masks, and topical body and facial treatments in spas surrounding the lake, and in cosmetic preparations marketed worldwide, but little is known about its antimicrobiological properties.
Methods We performed detailed microbial and chemical analysis of Dead Sea mineral mud compounded in dermatological and cosmetic preparations.
Results Using conventional bacteriological media (with or without salt augmentation), we found surprisingly low numbers of colony-forming microorganisms in the mud. The highest counts (up to 20,000 colonies per gram, mostly consisting of endospore-forming bacteria) were obtained on sheep blood agar. Test microorganisms (i.e. Escherichia coli, Staphylococcus aureus, Propionibacterium acnes, Candida albicans) rapidly lost their viability when added to the mud. Zones of growth inhibition were observed around discs of Dead Sea mud placed on agar plates inoculated with Candida or with Propionibacterium, but not with Staphylococcus or Escherichia.The effect was also found when the mud was sterilized by gamma irradiation. Using 35S-labeled sulfate as a tracer, bacterial dissimilatory sulfate reduction could be demonstrated at a low rate (0.13 ± 0.03 nmol / cm3.d).
Conclusion The antibacterial properties of Dead Sea mud are probably owing to chemical and / or physical phenomena. Possible modes of antimicrobial action of the mud in relation to its therapeutic properties are discussed.
Introduction
The Dead Sea, located at the lowest point of the Syrio-African Rift Valley, is a unique chemical, microbiological, and climatological environment.1–4 Its surface is currently 417 m below mean sea level. The water of the lake has a salt concentration of 340 g/ l; in order of decreasing concentration, its major cations are Mg2+, Na+, Ca2+, and K+, while Cl– and Br– are the main anions.
Resorts around the Dead Sea attract patients worldwide, who seek a cure for diseases such as psoriasis,5–7 atopic dermatitis,8 and rheumatic disorders.8,9 Treatments are based on two modalities: (1) bathing in Dead Sea water while the skin is exposed to solar radiation from which harmful short-wavelength UV radiation has been effectively filtered out, and (2) mud packs prepared from the highly saline, sulfide-rich, black mineral mud that is found abundantly in the area.9,10 Deposits of gray to black mud are located at the mouths of the mostly dry beds of the runoff streams that flow into the Dead
Sea (from the Judean Mountains in the west, the Jordanian Moab Mountains in the east, and the Jordan Valley in the north).11 These mud deposits are impregnated with sulfide-rich, anoxic subsurface salt-rich brines that emerge from groundwater, or with water from sulfide-containing hot springs found at the lake’s shores.12 The mud layers were formed in the Holocene era, less than 10,000 years ago. The mineral mud is also extensively used as an ingredient in cosmetic preparations.13 For thousands of years the Dead Sea has been known for its medicinal properties. The healing powers of Dead Sea water are mentioned in the Babylonian Talmud (Tractate Shabbat 108b) by Galen (122-c200) and by other old-world historians.14–16 The first chemical analysis of Dead Sea water was performed by Lavoisier in 1772 and published 4 years later.17 It is interesting to note that this was only the second documented quantitative analysis of any natural water source. The authors correctly recognized the predominance of alkaline “earth salts” over NaCl, the high concentration of Cl–, and the presence of both Ca2+ and Mg2+. The elements potassium and bromine were unknown at that time.17,18 In spite of extensive use of this gray or black mud in dermatological and cosmetic preparations, there is a paucity of information concerning its chemical composition, indigenous microbiological flora, and effect on pathogenic and nonpathogenic microorganisms. We therefore performed this study to gain a better understanding of the antimicrobiological properties of Dead Sea mineral mud.
Materials and Methods
Dead Sea mud samples
Crude (unprocessed) Dead Sea mud, supplied by Ahava – Dead Sea Laboratories Ltd, a commercial regional company that manufactures Dead Sea cosmetics, was collected at the
north-western end of the Dead Sea at a distance of 200 – 400 m from the shore. Samples were obtained at a depth of 40 – 60 cm below the surface. In a number of the experiments (as indicated later), the mud was processed by adding unsterilized tap water (18% by weight) to the crude mud; the samples subsequently were homogenized and filtered through a 300-mm sieve. Some of the unprocessed and processed samples were sterilized by gamma irradation (60Co, 2.5 MRad).
Mineralogical and chemical analyses
The mineralogical composition of the mud was determined by
X-ray diffraction (XRD). Elemental analysis (of the water-soluble and water-insoluble fractions) was performed using the inductive coupled plasma (ICP) method as described by Kafri et al.19 Water content was determined by drying 100-g samples of mud for 76 h at 105 °C until a constant weight was achieved. The pH of mud slurries (10 g of mud mixed with 50 ml of distilled water) was measured using a Beckman pH meter (Fulletron, CA, USA). In addition, we measured the pH of the supernatant fraction obtained following centrifugation of the mud for 10 min at 10,000 g. Sulfide was assayed by iodometric titration, according to the procedure 4500 – S2–F and 4500 SO32–C Phenanthroline Method,20 or following distillation of the sulfide with a stream of nitrogen (after weighed portions of mud were acidified),21 trapping the sulfide in 5 ml of 2% zinc acetate, and quantifying the sulfide colorimetrically using the methylene blue method.22
Microbial cultures and growth media
Cultures of Escherichia coli (ATCC 8739), Staphylococcus aureus (ATCC 6538), and Propionibacterium acnes (ATCC 11827), and Candida albicans (ATCC 10231) were routinely grown on tryptic soy agar (TSA, Difco, San Jose, CA, USA), aerobically at 30 °C. When indicated, inoculated plates were incubated anaerobically by placing them in sealed plastic bags in the presence of AnaeroGen sachets (Oxoid, Hampshire, UK).
The following growth media were employed for microbiological testing of mud samples, as recommended for the analysis of cosmetic products:23 (1) Modified Letheen Broth (MLB, Difco), a nonselective medium used to dilute cosmetic products for general counts of microorganisms; (2) modified Letheen Agar (MLA, Difco), a nonselective medium for counting microorganisms in cosmetic products; (3) Oxytetracycline-Glucose-Yeast extract Agar (OGYE Agar, Difco), for counting yeast and fungi in cosmetic products; (4) Tryptic Soy Agar ( TSA, Difco), a nonselective medium for general counts; (5) Sheep Blood Agar (SBA; CHAI Laboratories, Rehovot, Israel), which consists of TSA supplemented with 5% sheep blood, a nonselective medium for examination of the hemolytic capacity of microorganisms; (6) Plate Count Agar (Difco); (7) MacConkey Agar (Difco), a selective medium for enteric Gram-negative bacteria; (8) Cetrimide Agar Base (CAB, Difco), a selective medium for pseudomonads; and (9) Baird-Parker Agar (BP, Difco), a selective medium containing egg albumin, tellurite, glycine and pyruvate, designed for isolation of Staphylococcus aureus from cosmetic products. In some experiments, TSA, MLA and OGYE media were supplemented with 10% NaCl. Additional media used for microbial enumerations were: (10) yeast extract (2 g/ l), amended with different concentrations of NaCl and / or MgCl2·6H2O (up to 100 g/ l of each). At salt concentrations of up to 50 g/ l, the agar concentration used was 15 g/ l, and at higher salt concentrations this was increased to 20 g/ l; (11) Brewer thioglycolate medium (Difco), with or without NaCl or MgCl2 amendment, as indicated. Nine ml aliquots of this medium were dispensed into screw-capped test tubes; and (12) medium designed for extremely halophilic Archaea from the Dead Sea (such as Halorubrum sodomense) containing (g / l): NaCl, 125; MgCl2·6H2O, 160; K2SO4, 5; CaCl2·2H2O, 0.1; yeast extract, 1; casamino acids, 1; starch, 2; pH 7.0. This medium was used both in liquid form and in solidified form with 20 g/ l of agar.
Enumeration of microorganisms in Dead Sea mud
Portions (1 g) of mud were diluted with 10-ml sterile phosphate buffer (0.01 M KH2PO4, sometimes supplemented with 10% NaCl, adjusted to pH 7.0 with KOH) or in MLB, as indicated. Ten-fold dilutions were prepared in buffer, then 0.1-ml aliquots were spread evenly on the surface of the agar by means of a sterile plastic rod. Plates were incubated aerobically at 30 °C for up to 7 days.
Anaerobic incubation conditions were obtained by placing the plates in sealed plastic bags in the presence of AnaeroGen sachets (Oxoid, Hampshire, UK). For enumeration of fermentative anaerobes in Brewer thioglycolate medium, tenfold dilutions of mud were made directly in the tubes containing 9 ml of viscous liquid medium, and the results were examined for up to 2 weeks.
To test for survival of known microorganisms, mineral mud samples (20 g) were placed in sterile 100-ml plastic cups and mixed with a 2-ml suspension of the culture to be tested in sterile
0.25 M phosphate buffer, pH 7.0. Suspensions of E. coli, S. aureus and P. acnes were adjusted to an optical density of 0.1 at 550 nm; the suspension of C. albicans had an optical density of 1.0. After different incubation times at 30 °C in a moisture-saturated incubator, 1-g samples were withdrawn, 10-fold dilutions in sterile phosphate buffer were prepared as described earlier, and 100 ml aliquots were spread onto TSA plates in four replicates. Plates were counted after 3 days of incubation at 30 °C. In some of the experiments, plates for the enumeration of P. acnes were incubated under anaerobic conditions.
Measurement of bacterial dissimilatory sulfate reduction While avoiding air contact to the extent possible, six samples of Dead Sea mineral mud were collected in 5-ml syringes. After injection of 35S-sulfate (approximately 100 kBq), the six syringes were closed with butyl rubber stoppers. The syringes were then placed in a large bottle containing anoxic dithionite-reduced water (to make sure that no oxygen was present during incubation) and incubated in the dark at room temperature. Incubation was terminated by quickly transferring the samples to 5 ml of a 5% zinc-acetate solution. Three samples were fixed after 12 h and served as controls, while the remaining three were incubated for a further 56 h. After fixation, the samples were stored at -20 °C. After each sample was centrifuged (10 min, 3000 g), the supernatant was removed and the sulfate concentration was measured on a Sykam ion chromatograph system equipped with a polystyrene-based LAC A14 column and a S3111 detector (Sykam, Gewerbering Eresing, Germany). The samples were distilled according to the single-step chromium reduction method of Fossing and Jørgensen.24 Finally, the amount of radioactivity in the supernatant and the pellet (i.e. the sulfate and the sulfide) was quantified in a liquid scintillation analyzer (Tricarb, Wellesley, MA, USA).
Antimicrobial activity of Dead Sea mud Antimicrobial activity of Dead Sea mud was tested on TSA plates on which suspensions of test organisms (0.1 ml of E. coli, P. acnes or S. aureus in 0.25 M phosphate buffer, pH 7.0 at OD 550 of 0.01 or 0.001; OD 550 of 0.1 for C. albicans) were evenly spread. Discs of 0.1 ml of Dead Sea mud were prepared by filling sterile plastic 1-ml syringes with mud, and using the piston to press out 0.1-ml portions; a sterile knife was then used to cut the discs. Three hours after inoculation with the test organism, up to 4 – 5 discs were placed on the surface of the agar plates and incubated for 36 h at 30 °C, and the appearance of inhibition zones around the discs was recorded.
Results
Chemical analyses
The consistency of Dead Sea mineral mud is that of fine clay. Its mineralogical composition is presented in Table 1. The crude mud was 35.6% water, as determined by measuring the difference in weight of the mud before and after it was dried at 105 °C. The density of the mud was 1.67 g/ cm3, and the pH of the slurry sediment was 6.4. Table 2 shows the results of the chemical analysis of crude mineral mud. The concentration of sulfate, as assessed in the course of the sulfate reduction experiments according to procedure 4500 – S2–F and 4500
Table 1 Semi-quantitative mineralogical composition of the clay fraction (< 2-mm particle size) of Dead Sea mineral mud (values in percentage by weight)*
Mineral Percent by weight
Illite–smectite phases 50 – 70
Kaolinite 10 – 20
Illite 10 – 15
Calcite 5 –15
Quartz 1 –5
Chlorite < 5
Palygorskite < 5
*Results are mean values of X-ray diffraction analyses of two separate aliquots of the same sample of crude mineral mud. One was analyzed after washing with distilled water, and the second after treatment with dilute HCl and H2O2. Analyses were performed at the Geological Survey of Israel, The Ministry of National Infrastructures, Jerusalem.
SO32–C Phenanthroline Method,15 was 3.1 mm. Sulfide was present in crude, processed and irradiated Dead Sea mineral mud in concentrations of approximately 700, 300, and 300 p.p.m., respectively. The content of organic matter was nearly 4%.
The results of our elemental analysis of the interstitial water fraction of the mud are summarized in Table 3. The interstitial water is hypersaline brine, with a salt concentration comparable to that of Dead Sea water, but differing in its ionic composition. Notably, the solution is higher in potassium and lower in calcium than Dead Sea water. Its pH was 5.6 – 5.7.
Microbial counts and sulfate reduction activity in Dead Sea mineral mud The numbers of colony-forming microorganisms recovered from the mud are shown in Table 4. The highest counts were consistently obtained on media of low salinity; inclusion of NaCl and/or MgCl2·6H2O, in concentrations of up to 150 g/ l, each greatly reduced colony recovery. Endospore-forming bacteria were most commonly encountered; Bacillus-type morphologies were found in aerobic media, and Clostridium-type cells in Brewer thioglycollate medium. No growth was obtained in a high MgCl2-high NaCl medium designed for Halorubrum sodomense and other halophilic Archaea found in the Dead Sea. The highest counts were obtained using sheep blood agar (up to 2 ´ 104 colonies per g of mud with aerobic incubation, 7 ´ 103 following anaerobic incubation). Other rich, nonselective media yielded only a few hundred colony-forming units per gram of mud. These values are several orders of magnitude lower than counts found in samples of common soils (results not shown). No growth was recorded on BP and cetrimide agar plates, designed for
Table 2 Dead Sea mineral mud (dry weight 77.5%) was analyzed by inductively coupled plasma (ICP AES and ICP MS) methods and wet analytical methods as described by Kafriet al.14 Analysis was performed at the Geological Survey, Jerusalem, The Ministry of National Infrastructures of The State of Israel, on 23.9.2003 by Dr Olga Yoffe and approved by Dr Ittai Gavrieli, Director of Geochemistry and Environmental Geology Division
Content in percentage Major minerals
20 SiO2
15.5 CaO
4.8 Al2O3
4.5 MgO
2.8 Fe2O3
1.7 Na2O
1.3 K2O
0.5 TiO2
0.4 SO3
0.3 P2O5
6.7 Cl
0.2 Br
Content in PPM Trace elements
550 Sr
250 Mn
200 Ba
75 Cr
60 Zn
60 V
40 Ni
25 Li
20 Cu
8 Co
4 Pb
3 Th
2 As
2 U
2 Mo
1 Sn
< 1 Ag
0.7 Be
0.6 Cd
0.2 Sb
the selective isolation of Staphylococcus aureus and Pseudomonas aeruginosa, respectively.
The rate of dissimilatory sulfate reduction, measured using 35S-labeled sulfate as a tracer, was 0.13 ± 0.03 nmol / cm3.d, indicating that some type of prokaryotic activity persisted in the mud.
Bactericidal effect of Dead Sea mud When suspensions of E. coli, P. acnes or C. albicans were mixed with Dead Sea mud, the number of colonies that could be recovered declined rapidly. After 3 days of incubation, limited numbers of C. albicans could be recovered, while
Table 3 Chemical analysis of the interstitial water of Dead Sea mineral mud. Crude Dead Sea mineral mud was centrifuged (10 min, 12,000 g) to extract the liquid phase. The liquid phase was analyzed by at the Geological Survey of Israel, The Ministry of National Infrastructures, Jerusalem, by inductive coupled plasma methods (ICP) as described by Kafri et al.14 The concentrations of major ions in Dead Sea water (mean values for 1996) are provided for comparison
Dead Sea water (1996) (mg/l) Interstitial water (mg / l) Macro-elements
230,400 140,000 Cl + Br
45,900 28,250 Mg
36,600 20,200 Na
17,600 9800 Ca
7800 9500 K
150 Sr
< 1000 SO4
mg/l Micro-element
9800 Mn
41.08 Ni
0.69 Co
2.14 Cu
16.0 Zn
0.2251 Y
0.132 Cd
0.1968 La
0.3198 Ce
0.02716 Pr
0.11439 Nd
0.02522 Sm
0.00836 Eu
0.00578 Tb
0.03936 Gd
0.03567 Dy
0.00787 Ho
0.01968 Er
0.00221 Tm
0.01292 Yb
0.00197 Lu
0.55 Pb
1.99 U
the numbers of E. coli and P. acnes had declined by 3 – 4 orders of magnitude (Fig. 1). In processed (diluted, filtered) mud, with or without radiation-induced sterilization, the decline was less pronounced. In another experiment, comparing the decline in viable numbers in unprocessed mud with or with- out radiation-induced sterilization, no significant differences were observed between the survival of organisms in sterilized vs. unsterilized mud (results not shown). There was only a slight decline in colony-forming numbers of S. aureus following exposure to Dead Sea mud. One possible explanation for the differences observed in survival between crude and processed mud is the difference in salinity. However, another potentially significant difference between the two types of mud is their concentrations of
Table 4 Numbers of colony-forming bacteria recovered per gram of crude Dead Sea mineral mud on different growth media incubated under different conditions. Portions (0.1 ml) of dilutions of Dead Sea mud in 10 mm phosphate buffer, pH 7.0, were streaked on agar plates, and incubated at 30 °C. Colonies were counted after 3 days. Numbers of colony-forming units are presented as mean values ± standard deviation (n = 3–4)
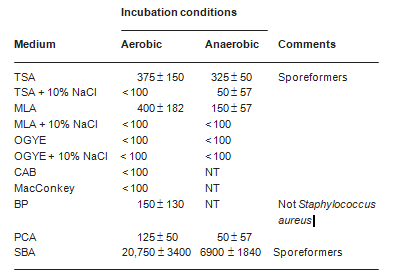
TSA = tryptic Soy Agar; MLA = modified letheen agar; OGYE = oxytetracycline-glucose-yeast extract agar; CAB = cetrimide agar base; BP = Baird-Parker agar; PCA = plate count agar; SBA = sheep blood agar; NT = not tested.
sulfide. Measured iodometrically, the sulfide concentration was 700 p.p.m. for crude mud, while diluted and irradiated mud contained only 300 p.p.m. sulfide (Fig. 1). The differ- ence was owing to oxidation of sulfide by oxygen in the dilu- tion water and in the air to which the mud had been exposed during the manipulations. To test whether the difference in sulfide concentration may explain the differences in observed survival, we examined the sensitivity of the test organisms to sulfide exposure. Tubes with 5 ml of TSB containing different sulfide concentrations (added as Na2S·9H2O), and controls without sulfide, were inoculated in triplicate with the test organisms; growth was recorded after incubation at 30 °C for 5 days. The minimal inhibitory concentrations of sulfide forC. albicans, A. niger, E. coli, P. acnes, and S. aureus were 64, 256, 1, 1, and 2 p.p.m., respectively. Although direct extrapolation of these results to the conditions prevailing in Dead Sea mud is not feasible (most sulfide there is bound and not freely dissolved), the importance of sulfide as a factor in the death of microorganisms in Dead Sea mud is strongly suggested by a correlation between the decline in viability of the different organisms (Fig. 1) and their sensitivity to sulfide. To further examine the nature of the antibacterial activity of Dead Sea mud, disks of 0.1 ml of mud or radiation-sterilized mud were placed on TSA plates inoculated evenly with suspensions of the test organisms. Inhibition zones were obtained
Table 5 Antimicrobial effect of discs of Dead Sea mud and radiation-sterilized Dead Sea mud on different microorganisms. Mud discs were placed on TSA plates inoculated evenly with suspensions of different microorganisms. After incubation for 2 days at 30 °C, the diameter of the inhibition zones around the discs was measured. The data presented are mean values of eight independent determinations
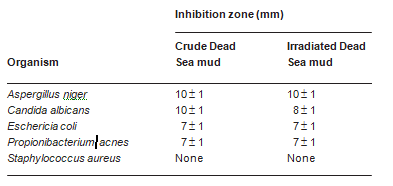
around the discs on plates inoculated with A. niger, C. albi- cans, E. coli, and P. acnes, but not with S. aureus (Table 5). The inhibition was probably not primarily owing to the diffusion of salt from the discs into the agar. When the discs were replaced with filter paper (5 mm in diameter) onto which solid NaCl (14 mg) or MgCl2·6H2O (27 mg) was added (in quantities calculated to be equivalent to those of salt present in the mud discs), no inhibition zones were observed. The effect is thus probably owing to the diffusion of toxic substances, such as sulfide, from the mud into the agar.
Discussion
Our chemical analyses of Dead Sea mud show a few prominent features. First, the mud is hypersaline, with the salt concentration of interstitial waters approaching that of Dead Sea water. However, the ionic composition of mud differs somewhat from that of Dead Sea brines. Other important features are a high content of organic matter (almost 2–3% by weight) supporting prokaryotic metabolism in the form of sulfate reduction, and the presence of both soluble and insoluble sulfur compounds. The latter finding is not surprising, consider- ing that brine emerging from hot springs and groundwater on the Dead Sea beach and the lake’s bottom contain sulfides. These sulfides were believed to have been produced by the activity of sulfate-reducing bacteria.12 However, we now have demonstrated that bacterial sulfate reduction still occurs within the mud at a very low rate (0.13 ± 0.03 nmol / cm3.d), a value several orders of magnitude less than found to occur in most marine sediments, but still significantly greater than the detection level of the method. Until quite recently, there have been indications that bacterial sulfate reduction also takes place in the bottom sediments of the Dead Sea,25 but currently there is little if any sulfate reduction activity in Dead Sea water.2,3 Little is known about the process of dissimilatory
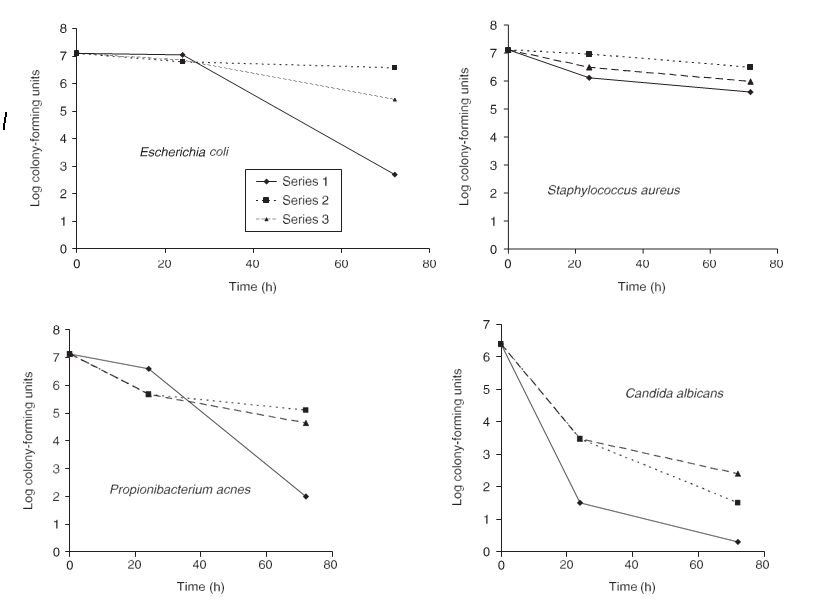
Figure 1 Survival of different microorganisms introduced to Dead Sea mineral mud. Suspensions of Escherichia coli, Staphylococcus aureus, Propionibacterium acnes, and Candida albicans (2 ml) were mixed with 20 ml of crude mud (◆), mud processed by the addition of 18% water and filtration (■), and mud processed by the addition of 18% water and filtration plus irradiation with 2.5 MRad of 60Co (▲). After different periods of incubation at 30 °C, samples were withdrawn, tenfold dilutions were prepared using saline, and the numbers of colony-forming units were determined on TSB agar plates
sulfate reduction in the bottom sediments of the Dead Sea. Prior to the 1979 overturn of the water column, the hypolimnion of the meromictic Dead Sea was anaerobic and con- tained sulfide. Stable isotope analyses showed that the sulfide was enriched in light sulfur isotopes relative to the sulfate, which points to bacterial sulfate reduction as the source of the sulfide.25,26 The microorganisms responsible for the formation of the sulfide have not yet been isolated, and we still do not know of any bacterium that is capable of performing dis-similatory sulfate reduction at the level of salinity encountered in the Dead Sea.26 Attempts to quantify sulfate reduction in Dead Sea sediments by following the formation of H235S from 35SO2– have never yielded conclusive evidence about the process (A. Oren, unpublished observations 1980–1990). Whether sulfate reduction has a direct impact on the therapeutic effect of this mud is unknown.
The discrepancy between the relatively large colony counts obtained on SBA and the significantly lower counts on MLA, TSA and the other media may be attributed to the presence of a dormant microbial population that does not form colonies even on rich, nonselective media, but can be activated by sheep blood. As most of the colonies that developed on SBA were endospore-forming bacilli, the dormancy could be related to a low efficiency of germination of bacterial endospores on the other media rather than to the impaired multiplication capacity of vegetative forms.27
A variety of nonpathogenic filamentous fungi have recently been isolated from Dead Sea water, but we elected not to study fungi. Some species may survive in the hypersaline brine for extended periods, probably as spores.28 Dead Sea black mineral mud is an anaerobic environment. We, therefore, tested for the presence of halophilic and
nonhalophilic anaerobic microorganisms in the mud. While sediments of the Dead Sea have been shown to contain specialized halophilic anaerobic bacteria, such as Halo-Bacteroides halobius,29 Sporohalobacter lortetii (formerly Clostridium lortetii),30 and Orenia marismortui (formerly Sporohalobacter lortetii),31 we did not find large quantities of such anaerobes. Counts in Brewer thioglycolate medium were a few hundred cells per gram of mud. Increasing the salt concentration of the medium further decreased the recovery of microorganisms, indicating that the organisms grown in the enrichments were not active in the mud in situ. Rather, they were present as spores and were activated by the shift to lower salinity in the media. In this respect, it should be remembered that the first microorganisms isolated from the Dead Sea in the last decade of the 19th century were tetanus and gas gangrene-causing bacteria which had survived in the mud as resistant endospores.32
The hostility of Dead Sea mud toward non halophilic microorganisms was shown by the rapid decline in the number of colony-forming units when E. coli, P. acnes, S. aureus and
C. albicans were mixed with the black mud. All our test organisms were facultative anaerobic or aerotolerant fermentative microorganisms. Therefore, this phenomenon cannot be explained by the anaerobic conditions prevailing in the mud. No significant difference was found in the killing of added organisms between radiation-sterilized and untreated mud. Hence, the possibility that biological factors (protozoa, bacteriophages) are responsible for the decline can thus be ruled out. The apparent bactericidal effect of Dead Sea mud may be owing to its high salt concentration combined with its special ionic composition. Exposure to Dead Sea water rapidly kills E. coli.33 A 17% solution of Dead Sea salts (“bath salts”) used as a substitute to bathing in the Dead Sea is also bactericidal to E. coli (Z. Ma’or, unpublished observations, 1985–1990). Another possibly important factor is the low pH (approximately 5.6) measured in the interstitial waters of the mud, which is less than that (pH 6.0) of the Dead Sea proper. The somewhat higher pH value of mud-distilled water slurries may be attributed to shifts in the carbonate system; it has been documented that the pH of Dead Sea water increases sharply upon dilution with distilled water.25 Bacteria such as E. coli also survive poorly in normal seawater (35 g/l of salt), probably owing to a variety of factors, including abiotic factors (light, salinity, pH, nutrient deprivation, and temperature) as well as biotic factors (grazing and competition, bacteriophages, antibiotics and toxins).34,35 The factor that may be most important is the presence of high concentrations of sulfide. The mud is rich in sulfide (derived from bacterial sulfate reduction that took place in the distant geologic past,12 as well as from sulfate reduction activity within the mud itself), as shown in this study. All test organisms were sensitive to sulfide; all minimal inhibitory concentrations measured were less than the actual sulfide concentrations measured in the mud. Although a comparison of these parameters is not straightforward, because most of the sulfide present in the mud is bound rather than freely dissolved, the immediate decrease in the total sulfide pool upon exposure to oxygen shows the presence of at least some highly reactive sulfide.The appearance of inhibition zones around discs of mud placed on a lawn of different test organisms was probably not owing to the presence of high salt concentrations in the mud, because the addition of equivalent amounts of NaCl or MgCl2 failed to cause the same effect. It is possible that diffusion of toxic sulfide was responsible for the formation of inhibition zones. No such zones were obtained when S. aureus was used as the test organism. As shown in Table 5, S. aureus is the least sulfide-sensitive of all organisms tested. It would be difficult to quantify the effect of sulfide using more controlled experiments because sulfide binds to components within the mud and is rapidly oxidized when exposed to molecular oxygen.
We conclude that Dead Sea black mud contains only very low numbers of viable microorganisms and that most of these are endospore-forming nonhalophilic bacteria. The mud has pronounced antimicrobiocidal action, which is probably owing to the combination of high salt and sulfide concentrations plus low pH. This anti-microbiocidal capacity, including the inhibitory effect on potential skin pathogens, might partially explain the therapeutic properties of the mud, and may explain the antiacne effect attributed to facial Dead Sea mud masks (Z. Ma’or, unpublished observations, 1998). Plant extracts such as Clinopodium vulgare L. and the essential oils of flora, such as Eucalyptus pauciflora and Ocimum gratissimum, as well as metabolites extracted from marine sponges, are included in a list of antimicrobial therapies.36 Catechol derivatives, caffeic acid, flavonoids and chlorogenic acid are among hundreds of naturally originated compounds reported to have antimicrobial properties.37 Further research is needed to fully understand the antimicrobial activities of Dead Sea mineral mud.
Acknowledgments
We thank Shlomit Mimran, Lily Mana and Rahel Elevi for their help with the experiments, and Ittai Gavrieli and his staff at the Geological Survey of Israel for the chemical analyses. This study was supported by a grant from The Dead Sea Research Center, Israel.
References
- Nissenbaum A. The microbiology and biogeochemistry of the Dead Sea. Microb Ecol 1975; 2: 139 – 161.
- Oren A. The microbial ecology of the Dead Sea. In: Marshall KC, ed. Advances in Microbial Ecology, Vol. 10. New York: Plenum Publishing Co., 1988: 193 – 229.
- Oren A. Microbiological studies in the Dead Sea: future challenges toward the understanding of life at the limit of salt concentrations. Hydrobiologia 1999; 205: 1 – 9.
- Oren A. Biological processes in the Dead Sea as influenced by short-term and long-term salinity changes. Arch Hydrobiol Spec Issues Adv Limnol 2000; 55: 531 – 542.
- Abels DJ, Kipnis V. Bioclimatology and balneology in dermatology: a Dead Sea perspective. Clinics Dermatol 1998; 16: 695 – 698.
- Abels DJ, Rose T, Bearman JE. Treatment of psoriasis at a Dead Sea dermatology clinic. Int J Dermatol 1995;
- 34: 134 – 137.
- Even-Paz Z. Dermatology at the Dead Sea spas. Isr J Med Sci
- 1996; 32: S11 – 5.
- Halevy S, Sukenik S. Different modalities of spa therapy for skin diseases at the Dead Sea area. Arch Dermatol 1998; 134: 1416 – 1420.
- Sukenik S, Buskila D, Neumann L, et al. Sulphur bath and mud pack treatment for rheumatoid arthritis at the Dead Sea area. Ann Rheum Dis 1990; 49: 99 – 102.
- Sukenik S, Buskila D, Neumann L, et al. Mud pack therapy in rheumatoid arthritis. Clin Rheumatol 1992; 11:
- 243 – 247.
- Rudel BZ. The Composition and Properties of the Dead Sea Mud. PhD Thesis. The Hebrew University of Jerusalem, 1993.
- Gavrieli I, Yechieli Y, Halicz L, et al. The sulfur system in anoxic subsurface brines and its implication in brine evolutionary pathways: the Ca-chloride brines in the Dead Sea area. Earth Planet Sci Lett 2001; 186: 199 – 213.
- Ma’or Z, Magdassi S, Efron D, et al. Dead Sea mineral-based cosmetics – facts and illusions. Isr J Med Sci 1996; 32:
- S28 – 35.
- Even-Paz Z, Shani J. The Dead Sea and psoriasis. Int J Dermatol 1989; 28: 1 – 9.
- Kottek SS. Medica Judaica: The Dead Sea in ancient Jewish sources. Isr J Med Sci 1996; 32: S38 – 39.
- Nissenbaum A. The Dead Sea – an economic resource for 10,000 years. Hydrobiologia 1993; 267: 137 – 141.
- Lavoisier AL. Mémoire sur l’usage de l’espirit-de-vin dans l’analyse des eaux minérales. Mem. Acad Royal Sci Paris 1776; 2: 555 – 563.
- Macquer PJ, Lavoisier AL, Sage BG. Analyse de l’eau du lac Asphaltite. Hist Acad Royal Sci 1781; 69: 72.
- Kafri U, Lang B, Halicz L, et al. Geochemical characterization and pollution phenomena of aquifer waters in northern Israel. Environ Geol 2002; 42: 370 – 386.
- Phenathroline Method & Iodometric Method. In: Standard Methods for the Examination of Water and Waste Water, 20th edn, 1998 American Public Health Association,
- 1015 Fifteenth Street, NW Washington, DC 20005-2605 segment.
- Jørgensen BB, Fenchel T. The sulfur cycle of a marine sediment model system. Mar Biol 1974; 24: 189 – 201.
- Cline JD. Spectrophotometric determination of hydrogen sulfide in natural waters. Limnol Oceanogr 1969;
- 14: 454 – 458.
- Hitchins AD, Tran TA, McCarron JE. Microbiological methods for cosmetics. In: FDA Bacteriological Analytical Manual, 8th ed, 1995: 23.01 – 23.011.
- Fossing H, Jørgensen BB. Measurement of bacterial sulfate reduction in sediments. Evaluation of a single-step chromium reduction method. Biogeochemistry 1989; 8: 205 – 222.
- Nissenbaum A, Kaplan IR. Sulfur and carbon isotopic evidence for biogeochemical processes in the Dead Sea ecosystem. In: Nriagu JO, ed. Environmental Biogeochemistry, Vol. 1. Carbon, Nitrogen, Phosphorus, Sulfur and Selenium Cycles. Ann Arbor: Ann Arbor Science Publishers, 1976: 309 – 325.
- Oren A. Microbiological studies in the Dead Sea: future challenges toward the understanding of life at the limit of salt concentrations. Hydrobiologia 1999; 405: 1 – 9.
- Henis Y. Survival and dormancy of bacteria. In: Henis Y, ed.
- Survival and Dormancy of Microorganisms. New York: John Wiley & Sons, 1987: 1 – 108.
- Kis-Papo T, Oren A, Wasser SP, et al. Survival of spores of filamentous fungi in Dead Sea water Microb Ecol 2003; 45: 183 – 190.
- Oren A, Weisburg WG, Kessel M, et al. Halobacteroides halobius General nov., sp. nov., a moderately halophilic anaerobic bacterium from the bottom sediments of the Dead Sea. System Appl Microbiol 1984; 5: 58 – 69.
- Oren A. Clostridium lortetii sp. nov., a halophilic obligately anaerobic bacterium producing endospores with attached gas vacuoles. Arch Microbiol 1983; 136: 42 – 48.
- Oren A, Pohla H, Stackebrandt E. Transfer of Clostridium lortetii to a new genus Sporohalobacter gen nov. as Sporohalobacter lortetii comb. nov. and description of Sporohalobacter marismortui sp. nov. Syst Appl Microbiol 1987; 9: 239 – 246.
- Loret ML. Research on the pathogenic microbes of the mud of the Dead Sea. Palest Expl Fund 1892: 48 – 50.
- Oren A, Vlodavsky L. Survival of Escherichia coli and Vibrio harveyi in Dead Sea water. FEMS Microbiol Ecol 1985; 31: 365 – 371.
- Sass E, Ben-Yaakov S. The carbonate system in hypersaline solutions: Dead Sea brines. Mar Chem 1977; 5: 183 – 199.
- Rosen Y, Belkin S. Survival of enteric bacteria in seawater.
- FEMS Microbiol Rev 2001; 25: 513 – 529.
- Fox C, Antimicrobials. Cosmet Toil 2002; 117: 45 – 62. 37 Pauli A. Antimicrobial properties of catechol derivatives.
- 3rd World Congress on Allelopathy. Japan: Tsukuba, 2002: 26 – 30.