Synopsis
The physical stability of Dead Sea mud mask formulations under different conditions and their rheological
properties were evaluated as a function of the type and level of thickeners, level of the humectant, incorporation of ethanol, and mode of mud treatment. Formulations were evaluated in terms of visual appearance, pH, moisture content, spreadability, extrudability, separation, rate of drying at 32°C, and rheological properties. Prepared mud formulations and over-the-shelf products showed viscoplastic shear thinning behavior; satisfactory rheological behavior was observed with formulations containing a total concentration of thickeners less than 10% (w/w). Casson and Herschel–Bulkley models were found the most suitable to describe the rheological data of the prepared formulations. Thickener incorporation decreased phase separation and improved formulation stability. Bentonite incorporation in the mud prevented color changes during stability studies while glycerin improved spreadability. Addition of 5% (w/w) ethanol improved mud extrudability, slightly increased percent separation, accelerated drying at 32°C, and decreased viscosity and yield stress values. Different mud treatment techniques did not cause a clear behavioral change in the fi nal mud preparation. B10G and K5B5G were labeled as “best formulas” based on having satisfactory physical and aesthetic criteria investigated in this study, while other formulations failed in one or more of the tests we have performed.
INTRODUCTION
The Dead Sea is the most concentrated hypersaline area in the world; it has an average
salinity of 370 g salt per kilogram of water, compared with the ocean’s average salinity of
35 g salt per kilogram of water (1). As the name indicates, this sea is devoid of life, except
for salt-tolerant halophilic anaerobic bacteria (2).
Potassium chloride, magnesium chloride, calcium chloride, sodium chloride, and their
corresponding bromides constitute the major salt components of the Dead Sea, comprising
about 98% on a dry weight basis (3). Dead Sea mud is a suspension of very fi ne clay particles in water (4); it is the natural sediment of solid mineral clays with an interstitial solution of inorganic salts and sulfi de
compounds that arise from microbiological activity (5).
It is characterized by its blackish color and the distinct sulfur smell (1). In addition, at 25°C, it has a pH of 6.4–7.6, density of 1.6–1.8 g/l, water content of 30–40%, nonpatho genic microbial content of less than 100 CFU/g, and 86–98% of particles with size less than 5 mm (5).
The therapeutic effect of Dead Sea mud is related to its high mineral content and ability, due to its black color, to retain heat for many hours (3). In general, Dead Sea mud is used as packs for the treatment of rheumatic disorders such as myalgias, neuralgias, and osteoarthritis; masks to treat cutaneous skin disorders such as acne, der matitis, psoriasis, xerosis, and eczema; and baths to treat lipodystrophy and cellulite(6,7). In addition, Dead Sea mud and salts have been widely used in cosmetic and personal care
products including lotions, masks, soaps, creams, and shampoos (1,3). The formulation and processing of Dead Sea products is not a simple undertaking,and should be tackled using valid scientifi c and technical approaches. For example, the formulation of cosmetic emulsions containing Dead Sea salts is known to give rise to production and stability problems and development scientists must take into consideration the maximum salt level that can be incorporated into a formulation without affecting stability, consistency, and liquid phase separation while maintaining effi cacy (8). On surveying the commercially available Dead Sea mud mask products in the Jordanian market and interviewing technical and quality staff of Dead Sea products manufacturers, we have identifi ed phase separation, stability issues as well as diffi culty in processing, and filling as the major problems in this product category. Rational use of formulation additives can improve the properties of mud formulations. These additives include thickeners that are expected to decrease phase separation of fi nished product, humectants that may be used to prevent dehydration, and ethanol that accelerates mud drying after application onto the skin. This study was initiated to evaluate the effects of commonly used additives in Dead Sea mud formulations as well as mud treatment techniques on the physical properties, rheology, stability, and aesthetic acceptance of the finished mud products.
MATERIALS AND METHODS
MATERIALS
Dead Sea mud was provided by Numeira Mixed Salts & Mud Company Ltd. (Amman,
Jordan), bentonite (Al2O3.4SiO2.H2O) was purchased from Vickers Laboratories Ltd.
(West Yorkshire, England), kaolin (Al2H4O9Si2) was purchased from Merck (Darmstadt,
Germany), Natrosol® 250 HHX (hydroxyethyl cellulose) was provided by Ashland Inc.,
(Wilmington, DE), and glycerin (99.5%) and ethanol pure (96%) were obtained from
AZ-Chem (Selangor, Malaysia).
MUD PREPARATION
Effect of additives. Formulations were prepared using different concentration levels of thickeners [kaolin at 5%, 7.5%, 10%, 15% (w/w), bentonite at 2%, 5%, 7.5%, and 10%(w/w), and Natrosol® 250 HHX at 0.05% (w/w)] while glycerin was used as a humectant in some formulations at 10% (w/w) level. Then, according to their aesthetics, ease of mixing and processing, and separation percentage, the most satisfactory formulas (shown in Table I) were chosen for further investigation. Thickeners were incorporated into the mud by slow addition, as dry powder, over 15 min with continuous mixing using a planetary mixer (Home Electrics TC-800, China 5.5 l capacity) at medium speed, then the whole preparation was left to mix for another 15 min to ensure homogeneity. When kaolin and bentonite were used in combination, kaolin was
added fi rst since it produced lower thickness than bentonite, and then bentonite was added. Humectants were reported to prevent dehydration of the fi nished mud products on aging (9). Thus 10% glycerin was added to some of the studied Dead Sea mud mask formulations. Glycerin was incorporated by mixing with the mud for 5 min before the addition of the thickener as per the abovementioned procedure.
Ethanol was used at two concentration levels [(1% and 5% (w/w)] in selected formulations (K15G, B10G, K5B5G, K5B5, and N0.05G). It was added as the fi nal component to the mud mask mixture and mixed for an additional 5 min. Five over-the-shelf Dead Sea mask products were selected to be studied parallel to our prepared formulations and were coded as RV, NC, BL, BS, and AQ. These samples were
evaluated by the same procedures and at the same stability conditions as the prepared
mud formulations. The Dead Sea mud used in the above formulations was used as received without treatment except for the manual removal of stones (untreated mud). Effect of mud treatment method. To investigate the effect of mud treatment method on the properties of Dead Sea mud formulations, the untreated mud was either dried in a tray
Table I Compositions of the Selected Dead Sea Mud Mask Formulations Evaluated in the Stability Study
Composition percent (w/w)
Formula codea | Mud | Bentonite | Kaolin | Natrosol | Glycerin |
K15G | 75 | — | 15 | — | 10 |
K10 | 90 | — | 10 | — | — |
B10G | 80 | 10 | — | — | 10 |
K5B5G | 80 | 5 | 5 | — | 10 |
K7.5B7.5G | 75 | 7.5 | 7.5 | — | 10 |
K5B5 | 90 | 5 | 5 | — | — |
N0.05G | 89.95 | — | — | 0.05 | 10 |
K10B2G | 78 | 2 | 10 | — | 10 |
level of the additive.
JOURNAL OF COSMETIC SCIENCE
oven (Philip Harris Ltd, Shenstone, England) at 60°C for 72 h followed by milling and sieving through a 1-mm sieve to produce “dry powder” grade or by autoclaving the mud for 15 min at 121°C to produce “sterilized mud” grade. Two formulas (K10 and K5B5G) were prepared using the treated mud (i.e., both dry
powder and sterilized mud grades). These samples were evaluated by the same procedures and at the same stability conditions parallel to untreated mud formulations.
STABILITY STUDY
Formulations were stored in plastic jars under the following conditions and testing
performed at the specifi ed time points:
—Room temperature 25°C; analysis after the 14th and 28th days of storage.
—Elevated temperature 45°C; analysis after the 14th and 28th days of storage.
—Freeze–thaw cycling -20°C/24 h and room temperature/24 h; analysis after two
temperature cycles.
The formulas were tested to provide a baseline value after 24 h of preparation to ensure complete hydration of the formulation. Samples stored at 45°C were left for 2 h at room temperature before testing and room temperature samples were considered as control samples. Samples were evaluated visually, and tested for spreadability, extrudability, percent moisture content (w/w), rate of drying [percent (w/w)] at 32°C, pH, separation percent (w/w),and rheological properties. Visual assessment. The visual appearance of all formulations evaluated during the study period included color, phase separation behavior, and any other distinct behavior. Spreadability. The parallel plate method (10) was used to evaluate spreading behavior using an in-house spreadability testing apparatus that consisted of two smooth wooden
slides to hold the mud samples and a pan to hold a weight during measurement as illustrated in Figure 1.
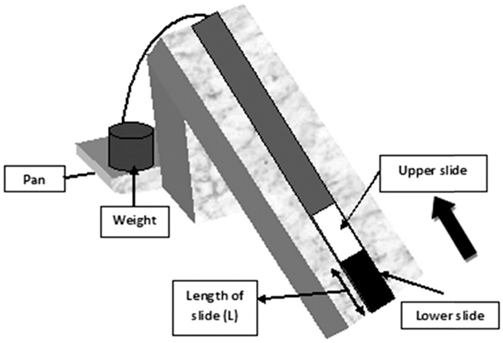
Figure 1. In-house spreadability testing apparatus used in the study.
PHYSICAL PROPERTIES AND STABILITY OF DEAD SEA MUD MASKS
Excess of the mud sample was spread on the surface of the lower slide, then the upper slide was used to cover the sample and a 100 g weight was used to compress the sample between the two slides for 2 min to obtain a layer of uniform thickness. The time (in seconds) required for the upper slide to move away to the edge of the lower one when the 100 g weight is placed on the pan was recorded and spreadability was calculated using to the following equation
S = m× l/t
where S is the spreadability (kg cm·s-1), m is the mass placed on the pan (0.1 kg), l is the length of the slide (cm), and t is the time (in seconds) required to move the upper slide (10). The test was performed in triplicates and data are presented as mean ± standard deviation (SD). Extrudability. The extrudability was evaluated using the method described by Purushothamraoet al. (11) after modifi cations. The test was performed using a clean pouch (PET/PE, 500 g capacity) with a tip opening diameter of 1 cm. The pouch was half-fi lled with the mud formulation, then for each formulation the extrudability was evaluated in triplicate by measuring the weight of mud ejected from the pouch opening on pressing with fingers, while holding the pouch in hands. The test was performed in triplicates and data are presented as mean ± SD.
Percent moisture content (w/w). Duplicate measurements of percent moisture content (i.e., Loss on Drying percent; LOD%) were performed using a moisture analyzer (Adam AMB 310; Adam Equipment, Kingston, United Kingdom). The test was performed on 1–2 g samples at 60°C until a constant weight was achieved, and the percent moisture content was calculated as percent (w/w).
% moisture content=initial weight final weight/initial weight× 100
The test was performed in triplicates and data are presented as mean ± SD. Drying rate at 32 °C. This test was performed to evaluate the drying behavior of different formulations under conditions mimicking the application of a mud mask onto human skin (12). Accurately weighed samples of about 1 g were spread on square pieces of aluminum foil (5 × 5 cm) using a spatula to get a uniform layer. Samples were then placed in a laboratory incubator (IN-010; Gemmy Industrial Corporation, Taipei, Taiwan) maintained at 32° ± 1°C to simulate human skin temperature and monitored for 20 min, which is the application time suggested by manufacturers of facial mud masks. Four samples were prepared from each tested formulation; one sample was withdrawn and weighed using an analytical balance (Shimadzu AY120 analytical balance; Nakagyo-ku, Kyoto, Japan) every 5 min up to 20 min. The drying percentage at each time point was calculated using the following equation
% drying=original weight weight after incubation/original weight×100%
JOURNAL OF COSMETIC SCIENCE
pH measurement. Mud dispersions [10% (w/w)] were prepared by adding deionized water to an accurately weighed amount of the tested formulation and leaving it to stir on a magnetic stirrer for 1 h (9). The pH of dispersions was measured using a microprocessor pH meter (Hanna pH 211; Woonsocket, RI). Separation percent (w/w). The liquid phase separation percent for different formulations was evaluated using the method described by Zague et al. (9). The test was performed, in triplicates, by placing 15–20 g of the tested formulations in 15-ml centrifuge tubes that were centrifuged at 3500 rpm for 15 min (K2042; Centurion Scientifi c Ltd., Chichester,United Kingdom). The liquid supernatant was decanted and weighed. The separation percent (w/w) was calculated using the following equation
Separation % w/w =weight of separated liquid phase/initial weight of sample×100
Rheological evaluation. The rheological properties of the mud formulations were studied using a Physica MCR 301 rheometer (Anton Paar, Austria) at 25°C using plate–plate system with a plate diameter of 25 mm and a gap of 1 mm. For each formulation, triplicate measurements of three different samples were performed for each of the rotational and oscillatory tests. In the rotational tests, fl ow curves and viscosity curves were recorded in the shear rate range of 0–100·s-1, then the obtained data were modeled using Casson and Herschel Bulkley models shown in equations (5) and (6). where τ is the shear stress, τc is the Casson yield point, γ is the shear rate, and ηc is Casson infinite shear viscosity, which is the limiting value of viscosity at infi nitely high shearrates (13).where τ is the shear stress, τHB is the Herschel–Bulkley yield point, k is the fl ow coefficient or consistency index, which serves as a viscosity index of the system, γ is the shearrate and the factor, n, is called the Herschel–Bulkley fl ow behavior index, which indicates
the shear thinning tendency (14).
The following parameters derived from rotational testing were evaluated to compare the
behavior of different formulations.
—Viscosity at different shear rate values (25/s and 75/s)
—Casson yield stress (Pa) and infi nite shear rate viscosity (Pa·s) from the Casson model.
—Consistency index (Pa·sn) and fl ow index (n) from the Herschel–Bulkley model.Oscillatory test was performed at a constant angular frequency of 10·s-1 to obtain rheo
grams describing the linear viscoelastic range (LVE). The following parameters derived
from oscillatory testing were used for evaluation and comparison purposes of the different
formulations (15).
PHYSICAL PROPERTIES AND STABILITY OF DEAD SEA MUD MASKS
—Yield stress (Pa) at the limit of LVE
—Storage modulus (G′, Pa) at the limit of LVE
—Loss modulus (G″, Pa) at the limit of LVE
—Flow point (Pa): the stress value when (G′ = G″).
—Damping factor: the ratio of loss modulus to elastic modulus (G″/G′)
Data analysis. Data were summarized as mean ± SD; the mean value and SD values were calculated for a duplicate or triplicate measurements according to the methodology used. Coefficient of determination (R2) was used to evaluate the parameters (regression coefficients) calculated from both rheological models (Casson and Herschel–Bulkley models)
RESULTS AND DISCUSSION
PREPARATION OF DEAD SEA MUD MASK FORMULATIONS
Thickeners can change plasticity and alter the application characteristics of the fi nished product and are useful in stabilizing the dispersion of solids and preventing gradual phase separation, which is occasionally observed during the shelf life of clay masks (9). Dead Sea mud mask formulations were prepared using three different thickeners (bentonite, kaolin, and Natrosol® 250 HHX), which were added to the mud in powdered form. This mode of addition was used as a way to disperse the thickener within the mud without having to increase its water content.
CHARACTERIZATION OF DEAD SEA MUD MASK FORMULATIONS
Visual assessment. Visual assessment results are described in Table II; samples varied in color from brown to dark gray and their consistency and thickness varied according to composition from easily extrudable formulations to thick formulations that were not easily extrudable. Natrosol® 250 HHX produced samples with a gritty texture, which may be attributed to the incorporation technique used for slow addition and mixing of Natrosol® as dry powder to the mud, which may have been inadequate to cause effi cient hydration and gelling of polymeric thickener. Separation percent (w/w). Separation percent decreased for all prepared formulas as compared with the untreated mud except for B10G and N0.05G, which showed separation values comparable to the untreated mud (Figure 2). Separation percent for over-the-shelf formulations was generally higher than that of the in-house formulations. In comparison to the untreated mud, separation percent of over the-shelf products was lower in the case of BL and RV only, while AQ, BS, and NC showed higher separation percent than the untreated mud. Spreadability. Glycerin has been shown by our results (Figure 2) to be essential for formulation spreadability; formulations prepared without glycerin, K10, and K5B5 were not spreadable using the spreadability testing procedure used in this study (no movement using the 0.1 kg weight) as can be seen from the results in Figure 2.
TableII
Results of Visual Assessment, Spreadability, and Separation Tests for Formulations at Initial Time Point
Formula codea Visual assessment | Spreadability (Kg cm·s-1) | Extrudability (g) | Separation percent (w/w) | |
Over-the-shelf samples | ||||
RV Faint brown color, thick texture, not easily extrudable | 0.008 ± 0.001 | 4.10 ± 0.17 | 4.20 ± 0.35 | |
BL Dark gray and brown (nonhomogenous color), not easily extrudable | 0.082 ± 0.011 | 3.45 ± 0.12 | 6.31 ± 0.29 | |
NC Dark gray color, easily extrudable | 0.181 ± 0.006 | 6.02 ± 0.33 | 9.84 ± 0.45 | |
BS Faint brown color, easily extrudable | 0.679 ± 0.186 | 4.75 ± 0.30 | 10.13 ± 0.11 | |
AQ Dark gray and brown (color variation), easily extrudable | 0.963 ± 0.064 | 7.94 ± 0.54 | 13.57 ± 0.27 | |
Stability samples | ||||
Untreated mud Gray-brown color, easily extrudable | 0.053 ± 0.008 | 6.63 ± 0.12 | 8.85 ± 0.02 | |
K15G Gray-brown color, thick, not easily extrudable | 0.025 ± 0.003 | 5.40 ± 0.16 | 2.98 ± 0.41 | |
K10 Gray-brown color, very thick, not easily extrudable | No movement using the 100 g weight | 6.61 ± 0.23 | 2.45 ± 0.49 | |
B10G | Dark gray color, easily extrudable | 0.217 ± 0.043 | 9.17 ± 0.38 | 8.02 ± 0.52 |
K5B5G | Dark gray color, easily extrudable | 0.099 ± 0.010 | 5.61 ± 0.27 | 4.99 ± 0.36 |
K7.5B7.5G | Dark gray color, easily extrudable | 0.054 ± 0.007 | 14.25 ± 0.53 | 5.34 ± 0.47 |
K5B5 | Gray-brown color, thick, and not easily extrudable | No movement using the 100 g weight | 6.52 ± 0.21 | 4.26 ± 0.95 |
N0.05G | Dark gray, gritty texture, acceptable extrudability | 0.180 ± 0.022 | 7.66 ± 0.34 | 7.03 ± 0.88 |
K10B2G | Dark gray color, thick, and not easily extrudable | 0.041 ± 0.004 | 5.63 ± 0.21 | 4.05 ± 0.45 |
aK = kaolin, B = bentonite, N = Natrosol® 250 HHX, G = glycerin, over-the-shelf brands = RV, NC, BL, BS, and AQ.
PHYSICAL PROPERTIES AND STABILITY OF DEAD SEA MUD MASKS
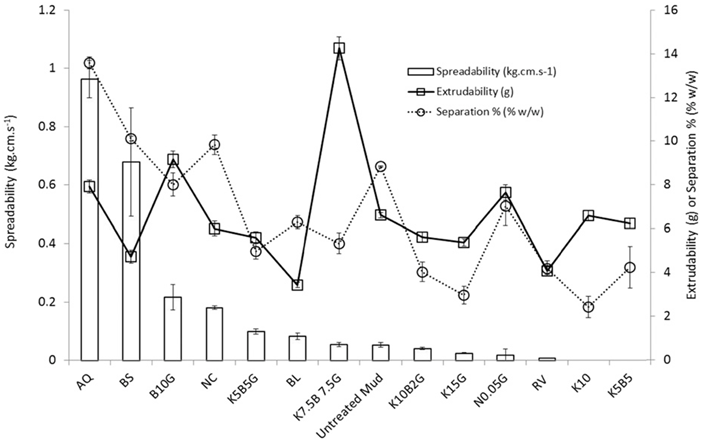
Figure 2. Separation percent, spreadability, and extrudability of tested samples at initial time point.
Results represent mean ± SD (n = 3). K = kaolin, B = bentonite, N = Natrosol® 250 HHX, G = glycerin,
over-the-shelf mud = RV, NC, BL, BS, and AQ.
Most formulations showed an improvement in their spreadability compared with untreated mud as shown in Figure 2. The best spreading behavior was for over-the-shelf products AQ, BS, and NC and the in-house B10G, K5B5G, and N0.05G. The spreadability ranking results were in agreement with subjective assessments. Extrudability. Extrudability values are shown in Figure 2. The extrudability is an indica
tor of the amount of mud that the customer can eject from the pouch container. No correlation was found between ease of spreadability and the ejected amount; the samples that were thick and not spreadable resulted in extruded amounts comparable to those formulas that were easily spreadable.
Drying rate at 32 °C. The water loss of formulations at 32° ± 1°C was monitored over a period of 20 min after application onto an inert surface. The time was set based on usage instructions stated on the over-the-shelf mud products and the temperature was chosen to simulate that of the skin. The duration of the test was chosen based on the instructions for use in the label of the over-the-shelf products stating that the product is to be applied for 15–20 min on the skin before washing it. Untreated mud showed a mass loss of 10% after 5 min and 20% after 10 min and did not
dry further until the end of the test (20 min). Most formulations showed a drying per
centage after 20 min comparable to that of the untreated mud. However, the rate of dry
ing was slower, which could be attributed to the presence of the thickeners and the
humectant.
Over-the-shelf products RV and BS showed the highest drying percentage after 20 min
(28%), which could be explained by the fact that they were the only over-the-shelf prod
ucts listing ethanol as a component.
JOURNAL OF COSMETIC SCIENCE
Percent moisture content (w/w). It was noted that the percent moisture content (w/w) was not
affected by changing the formulation composition; the values were always comparable to
that of the untreated mud. Literature data state that the percent moisture content (w/w) (LOD%) of Dead Sea mud at 25°C ranges from 30% to 40% (5). The percent moisture content (w/w) of our preparations ranged from 32% to 37%, and the untreated mud had a value of 35%. On the other hand, over-the-shelf Dead Sea mud products had a percent moisture content (w/w) range of 34–41% and the highest values observed for RV and NC products. pH. The pH value of the Dead Sea mud at 25°C was reported by Ma’or et al. as 6.4–7.6 (5). Our results show that the untreated mud had a pH of 8, whereas the pH of our formulations varied between 7.9 and 8.3. This slight difference in pH value could be attributed to the differences in the chemical composition of different mud samples collected from different sites. Rheological evaluation. The flow curves were used to calculate the values of yield stress, viscosity, flow index, consistency index, flow point, storage modulus, and loss modulus of each tested formulation. The effect of additives on the different rheological parameters was investigated. Over-the-shelf brands were evaluated using the same methodology and characterized in terms of their rheological parameters. The rheological results are summarized in Table III. Most samples showed a typical viscoplastic, shear-thinning behavior. A typical behavior of formula B10G is shown in Figure 3. Such a shear thinning or thixotropic behavior, in which the material will decrease in viscosity as the shear rate is increased or with progression of the shear time, decreases the load on machines during mixing and causes the material to flow easily during filling. In addition, thixotropic behavior will ensure efficient spreadability on skin and structural
consistency regain after application (3), which contributes to consumer aesthetic acceptance.
However, the rheological behavior of formulations K15G, K7.5B7.5G, K10B2G, NC, and RV was different; they showed a shear-thinning viscosity curve, while the flow curve behavior was not compatible with any of the known non-Newtonian-material fl ow curves as depicted in Figure 4 showing the behavior of formulation K7.5B7.5G as an example. Flow curves were fitted to the Casson and Herschel–Bulkley models. These models are considered the most commonly used models for time-independent flow behaviors with yield stress (16). It has been suggested that the rheological behavior of clay water suspensions is best described using the Herschel–Bulkley model (17). The Herschel–Bulkley model has been used by Abu-Jdayil and Mohammed (3) to study the effect of temperature and time on the rheological properties of a Dead Sea mask formulation. This could be explained by considering the fact that the quality of the regression and fitting using the Herschel–Bulkley model is expected to be better than that obtained using the Casson model due to the presence of a larger number of regression parameters (three in the Herschel–Bulkley model compared to two in the Casson model) (14,16,18,19). In addition, the Casson model makes an assumption that the flow behavior index or exponent is a constant equal to “0.5.
PHYSICAL PROPERTIES AND STABILITY OF DEAD SEA MUD MASKS
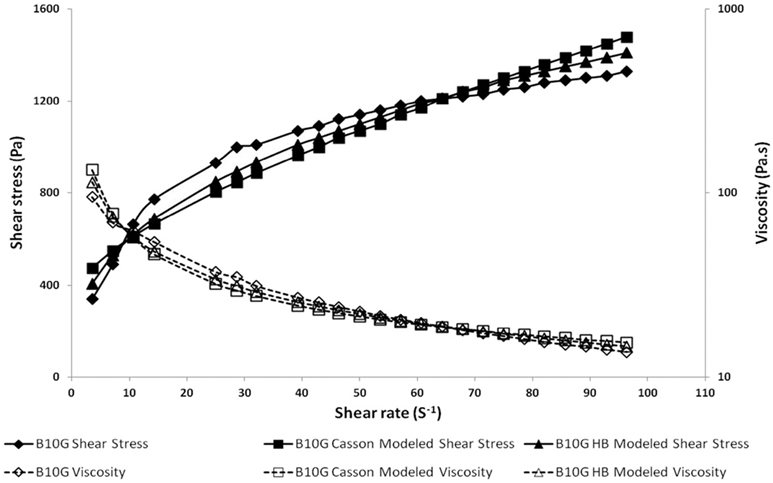
Figure 3. Typical shapes of Casson and Herschel–Bulkley (HB) modeled flow curves, data shown are for
B10G at the initial time (first day) point compared with the unmodeled flow of the same formulation sample.
However, when our data were fi tted to the Herschel–Bulkley model, we faced a problem
of meaningless negative yield stress values for many of the samples, which include AQ,
BL, BS, NC, K15G, K7.5B7.5G, K5B5, N0.05G, and K10B2G
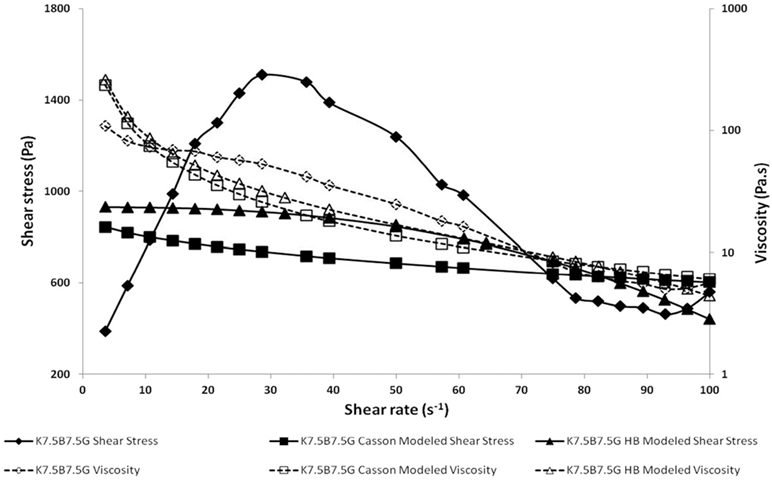
Figure 4. Casson and Herschel–Bulkley (HB) modeled flow curves for K7.5B7.5G at the initial time point compared with observed flow curves of the same formulation.
JOURNAL OF COSMETIC SCIENCE
Several procedures can be followed to overcome the problem of negative meaningless
yield stress values (18), the most applicable one for our samples involves using the Casson
model to calculate yield stress for all samples and incorporated the calculated yield stress
to the Herschel–Bulkley model and used it to fi t the data to calculate both consistency
and fl ow indices.
All formulations fi tted to both models with high R2 values except K15G,K7.5B7.5G, K10B2G, RV, and NC, which had negative meaningless values for all or some of the parameters; yield stress value (either Casson, Herschel–Bulkley, or both), consistency index, flow index, and infi nite shear viscosity. The behavior of K15G, K7.5B7.5G, and RV did not fit either model, whereas that of NC fi tted to Casson model only and K10B2G fitted to Herschel–Bulkley model only as shown in Table III. Correlating this deviated behavior with formulation composition revealed that the maximum level of thickener concentration that can be incorporated into a mud formulation without producing a deviation from the ideal shear thinning flow curves is 10% of either one thickener (kaolin and bentonite) or a combination of the two of them.
Casson yield stress values for our formulations were higher than that of the over-the-shelf samples; the highest was that of N0.05G. All in-house prepared formulations mentioned in Table I had higher Casson yield stress compared with untreated mud, except K5B5G. While only BL from over-the-shelf products had higher value than untreated mud. Yield stress is the minimum shear stress required to initiate material flow. It is an important determinant of product stability and the ease of product application by the end
user (20). Higher yield values ensure that the material will preserve its structure and consistency
and maintain particles in the medium with minimal sedimentation (20). On the other hand, lower yield stress guarantees easier distribution of a semisolid on the skin and easier extrusion out of its tube (21).
An optimum yield stress value is required to balance between the ability to pump and fill the product and the stability during transfer and storage (3). Consistency index values were comparable for most of our stability samples (300 400 Pa·sn), the highest was that of N0.05G and K10 and the lowest was that of K5B5.All in-house prepared formulations included in the stability study had higher values of
consistency index compared with the untreated mud, except K15G and K7.5B7.5G for which could not calculate the consistency index. The consistency index is an indicator of structural strength and serves as an index of the viscosity of the system (14), and when the formulation consistency index is higher, the
formulation viscosity, thickness, and yield stress are higher and the lower spreadability is
lower. The consistency index of the prepared in-house formulations was higher than that of the
over-the-shelf products; the lowest value was for AQ brand. Flow index values, an index of the shear thinning tendency of the samples (14), were less than one for all tested samples, which is an indicator of a shear thinning behavior (13). Viscosity value differences were more obvious at lower shear rate (25 s-1) than higher shear rate (75 s-1). The highest viscosities were for K15G, K10B2G, K7.5B7.5G, and K10, while
over-the-shelf products had lower values.
PHYSICAL PROPERTIES AND STABILITY OF DEAD SEA MUD MASKS
Oscillatory test parameter values include yield stress at LVE limit, storage modulus, loss modulus, and flow point were generally higher for our in-house formulations than NC, BS, AQ, and BL of the over-the-shelf products, while RV had values comparable to in-house formulations. Highest values were for K10, K5B5, and N0.05G, while the lowest were for AQ and NC. The storage modulus (G′) is the energy stored per unit volume, which is proportional to elastic component magnitude in the system contributed by cross-linking and/or aggregation, while the loss modulus (G″) is the energy dissipated per unit volume, which is
proportional to the extent of the viscous component contributed by the liquid-like portions. The ratio of G″/G′ indicates the strength of interaction of the internal structure and it is called the damping factor (20).
The values of the storage modulus were higher than those of the loss modulus for all tested samples, which is a property of elastic systems. The ratio of loss modulus to storage modulus, expressed as the damping factor, was comparable for all samples (ranging from 0.21 to 0.29). Higher formulation elasticity (higher storage modulus values) indicates a higher formulation thickness and yield stress and, consequently, a lower spreadability. Shear stress and storage modulus values for in-house samples were all higher than the untreated mud, except for B10G. On the other hand, over-the-shelf products had lower
values than the untreated mud, except for RV and BL, which had higher values.A flow point is the stress value when G′ = G″, which indicates the stress that will cause structural destruction of the material and consequent material flow (15). The higher the flow point, the higher stress required to cause material destruction, which could indicate higher product stability over its shelf life. On the other hand, it indicates higher yield stress values and lower spreadability. Only NC and AQ had lower flow point values than untreated mud, the remaining over the-shelf products and all of the in-house formulations had higher values than untreated mud
STABILITY OF DEAD SEA MUD MASK FORMULATIONS ON EXPOSURE TO STRESS CONDITIONS
The behavior of formulations listed in Table I and stored in plastic jars was investigated on exposure to elevated temperature of 45°C for a period of 4 weeks (accelerated stability study conditions) and freeze-thaw cycling. Samples stored at room temperature (25°C) were used as control samples. Appearance. During stability studies, some formulations showed changes in its appearance at different storage conditions and different time points. Two samples from over-the-shelf products (AQ and BL) had color changes to rusty red after 2 weeks of storage at both room temperature and accelerated conditions, which increased more after 4 weeks at the same conditions. On the other hand, only one formulation (K10) showed color changes to brown and rusty red at room temperature conditions after 4 weeks, and a similar behavior was shown at accelerated conditions, but started after 2 weeks of storage, a rusty red color was observed.
JOURNAL OF COSMETIC SCIENCE
Formulations K15G, K5B5, N0.05G, and K10B2G showed no color changes at room temperature, while color changes appeared at accelerated conditions after 2 weeks of storage; slight brown color appeared that changed after 4 weeks at the same conditions into a rusty red color for formulations K15G and K5B5 and remained brown for the other two formulations. In addition, K5B5G started color changes to a very slightly brownish color after 4 weeks at only accelerated storage conditions. Untreated mud showed a rusty red color after 4 weeks of storage at room temperature while the same changes started after 2 weeks of storage at accelerated conditions. It was noticed that color changes occurred in formulations without hydrated aluminum silicate thickeners (kaolin and bentonite) and those containing kaolin as the sole thickener. The presence of bentonite alone or combined with kaolin at the same concentration
level reduced the degree of color changes. This could be related to the high adsorption capacity of these materials that could bind whatever species responsible for color development, which may include iron compounds or carotenoids from Dunaliella (22). Separation percent (w/w). The separation percent test that was used as a tool in formulation screening at the initial development stages (13) was also used to follow up changes throughout stability study. Signifi cant changes in separation percent indicate early product failure on the shelf after marketing. The most acceptable formulations at initial time point were NC, BS, BL, B10G, K5B5G, K7.5B7.5G, and N0.05G, which were characterized by moderate separation percent (5–10%). AQ was the only one formulation that has extensive separation (>10%), whereas RV,
K15G, K10, K5B5, and K10B2G resulted in minimal separation percent; however, they were thick and not easily spreadable. Separation percent was affected by storage at different conditions, the behavior of most samples was a decrease in separation percent during stability at both room temperature and accelerated conditions, but it was more notable at accelerated conditions; all samples showed a decrease in separation percent at accelerated conditions, which was also notable in visual assessment of samples as a change in product texture to a thicker one. The decrease in separation percent most probably attributed to the loss of water from the formulations during storage. Separation behavior after freeze-thaw testing was not clear; some samples showed an increase in separation percent (RV, NC, BL, K15G, K10, K5B5G, and K10B2G), whereas the remaining formulations (BS, AQ, B10G, K7.5B7.5G, K5B5, and N0.05G) showed a decrease in separation after freeze–thaw cycles. pH values ranged from 7.93 to 8.32 at initial time point and during stability study there was slight variation in pH values (maximum pH change was 0.3 units).
Rheological evaluation. Flow curves of K15G, K7.5B7.5G, K10B2G, NC, and RV remained deviated from typical behavior at all the time points and different stability conditions, similar to their behavior at initial time point. The main change in Casson yield stress values during stability was the notable increase at
accelerated conditions, while only a slight change was noticed at room temperature storage. Some samples, BL and N0.05G, showed a decrease in yield stress on storage under accelerated conditions. In addition, viscosity and oscillatory test parameter values increased during stability study at accelerated conditions